Cardiorespiratory Fitness Associates with Cerebral Vessel Pulsatility in a Cohort Enriched with Risk for Alzheimer’s Disease
Abstract
Background:
There is increasing evidence that vascular disease risk factors contribute to evolution of the dementia syndrome of Alzheimer’s disease (AD). One important measure of cerebrovascular health is pulsatility index (PI) which is thought to represent distal vascular resistance, and has previously been reported to be elevated in AD clinical syndrome. Physical inactivity has emerged as an independent risk factor for cardiovascular disease.
Objective:
This study aims to examine the relationship between a measure of habitual physical activity, cardiorespiratory fitness (CRF), and PI in the large cerebral vessels.
Methods:
Ninety-two cognitively-healthy adults (age = 65.34±5.95, 72% female) enrolled in the Wisconsin Registry for Alzheimer’s Prevention participated in this study. Participants underwent 4D flow brain MRI to measure PI in the internal carotid artery (ICA), basilar artery, middle cerebral artery (MCA), and superior sagittal sinus. Participants also completed a self-report physical activity questionnaire. CRF was calculated using a previously-validated equation that incorporates sex, age, body-mass index, resting heart rate, and self-reported physical activity. A series of linear regression models adjusted for age, sex, APOE4 status, and 10-year atherosclerotic cardiovascular disease risk were used to analyze the relationship between CRF and PI.
Results:
Inverse associations were found between CRF and mean PI in the inferior ICA (p = .001), superior ICA (p = .035), and basilar artery (p = .040). No other cerebral vessels revealed significant associations between CRF and PI (p≥.228).
Conclusions:
Higher CRF was associated with lower PI in several large cerebral vessels. Since increased pulsatility has been associated with poor brain health and reported in persons with AD, this suggests that aerobic fitness might provide protection against cerebrovascular changes related to the progression of AD clinical syndrome.
INTRODUCTION
Alzheimer’s disease (AD), the most common form of dementia, is characterized by the accumulation of amyloid-beta plaques and neurofibrillary tangles [1, 2]. However, there is increasing evidence that vascular dysfunction may contribute to the evolution of the dementia syndrome of AD. Research shows that vascular disease risk factors such as hypertension, atherosclerosis, and smoking are all risk factors for developing a degenerative dementia like AD [3–6]. Further more, clinical cohort studies suggest that AD pathophysiolgical changes are detectable years before a diagnosis of mild cognitive impairment or AD dementia. This preclinical phase of AD provides a crucial opportunity for therapeutic intervention as recent clinical trials show that interventions applied earlier in the course of AD are more likely to achieve disease modification [7]. In an autopsy study of more than 1,100 older adults with and without dementia, researchers observed severe cerebral atherosclerosis and arteriosclerosis in approximately one third of participants which independently increased their risk of developing AD dementia and was associated with lower cognitive functioning [8]. These findings were independent of any infarct or AD pathologic effects. Another study, utilizing MRI imaging to examine cerebrovascular pathologies (CVP) and AD pathologies (ADP) in 457 older adults, revealed that the rate of cognitive decline was higher in persons with both CVP and ADP than those with only CVP, only ADP, or neither [9].
One important measure of cerebrovascular health is pulsatility index (PI). PI is used as a surrogate to assess vascular resistance and can be thought of as a measure of distal (downstream) resistance to flow [10, 11]. It is an intrinsic property of the vascular system, driven by the resistance differential across an artery, that enables stored energy in the elasticity of arteries to propagate throughout small-vessel blood circulation [12]. In previous research, PI measurements were acquired through the use of a Transcranial Doppler (TCD) [13–15]. TCD is a noninvasive, real-time measure of cerebrovascular hemodynamics and blood flow characteristics. However, advances in MR hardware and data acquisition have made assessing cerebrovascular hemodynamics much more clinically feasible, reproducible, and robust. Phase Contrast Vastly undersampled Isotopic PRojection (PC VIPR) is a specific 4D MRI flow scan that is particularly well suited for tackling the high-resolution demands of intracranial cerebral vessels. PC VIPR utilizes radial undersampling which creates accelerated acquisitions with small voxel sizes [16–20]. Post scan completion, hemodynamic measurements of PI can be performed in the cerebral blood vessels.
With increasing evidence that vascular disease risk factors may contribute to the occurrence of the dementia syndrome of AD, it is important to examine the relationship of cerebrovascular changes in AD to modifiable vascular risk factors. Lack of engagement in physical activity is an independent risk factor for cardiovascular disease [21]. Cardiorespiratory fitness (CRF) constitutes the physiological nexus of the effects of habitual physical activity, and reflects the overall capacity of the cardiopulmonary system [22, 23]. Accumulating evidence shows that higher levels of CRF are related to better brain health [24–27], lower risks of cardiovascular disease [28] and AD [29–31] in older adults. However, its relation to indices of cerebral hemodynamics, such as PI, has not yet been fully explored. Therefore, the aim of this study is to examine the association between CRF and vessel PI in a middle-aged cohort enriched for AD risk factors. We hypothesized that higher CRF would be associated with lower PI.
MATERIALS AND METHODS
Participants
Ninety-two cognitively unimpaired adults from the Wisconsin Registry for Alzheimer’s Prevention (WRAP) participated in this study. The WRAP is a longitudinal registry of approximately 1,500 middle-aged adults who were cognitively healthy and between the ages of 40 and 65 years at study entry [32]. Unimpaired cognition was adjudged via a diagnostic consensus conference, and based on intact performance on a comprehensive battery of neuropsychological tests, absence of functional impairment, and absence of neurologic/psychiatric conditions that might impair cognition [32, 33]. The 92 participants involved in this report were selected based on having usable PC VIPR data as well as data necessary for computing CRF (see below). Similar to the larger WRAP cohort, this sample was enriched with participants who had a positive family’s history for AD (69.6%) and were apolipoprotein E4 (APOE4) positive (34.8%). The University of Wisconsin Institutional Review Board approved all study procedures and informed consent was obtained from all participants included in the study.
MR IMAGING PROTOCOL
Subjects were scanned using an 8-channel head coil on a 3T MRI system (MR750, GE Healthcare, Waukesha, WI). Phase-contrast MR imaging data with 3-directional velocity encoding were acquired with a radially undersampled 4D flow MR imaging sequence, i.e., PC VIPR. Scan parameters for the acquisition were as follows: velocity encoding = 80 cm/s, imaging volume = 22×22×22 cm3, isotropic spatial resolution = (0.7 mm)3, 14,000 projection angles, scan time ∼7 minutes, TR/TE = 7.4/2.7 ms, flip angle α= 10°, receiver bandwidth = 83.3 kHz, retrospective cardiac gating reconstructed into 20 cardiac phases using temporal interpolation [34]. Time-averaged magnitude and velocity data were generated with an offline reconstruction for all participants [19].
Flow analysis
Hemodynamic evaluation of velocity vector fields, extracted from the PC VIPR data sets, was completed using an in-house tool developed in Matlab (The MathWorks, Natick, MA) [35]. The tool automatically generated angiograms from the time averaged complex difference data by setting a global threshold at μ+ 4*σ using normal distribution fit results, mean, and standard deviation (μ,σ). A thinning procedure [36], which conserves structure, was completed on the binary angiograms for centerline generation and labeling. Orthogonal analysis planes were automatically generated for every centerline point using the unique centerline for each vessel. Interactive vessel selection was completed using a single click in the axial plane for the distal petrous ICA, the middle of the M1 segment of the MCA, 5 mm above the basilar artery origin, and 45 mm from the torcula/confluence of the superior sagittal sinus. Vessel ROI’s were generated using a k-means approach assuming a two-region model (1.background 2.vessels). Cerebrovascular flow features were computed using the vessel ROI’s and velocity vector fields. Specifically, pulsatility index (PI = (Qmax - Qmin)/Qmean ; Q = flow ) was calculated for all vessel segments. Measurements from the left and right inferior ICA, the left and right superior ICA, and the left and right MCA, were averaged to obtain mean inferior ICA, superior ICA, and MCA pulsatility values [20].
Obtaining PI measurements requires good cardiac gating which was difficult to obtain consistently for some participants. Furthermore, anatomical variances of vessels in the Circle of Willis (i.e. hypolplasias, bifurcations) can influence hemodynamic characteristics [37]. For these reasons, some vessels were unsuccessfully segmented, resulting in missing PI values for specific vessels within participants. Of our 92 participants, 92 had usable ICA data, 75 had usable basilar artery data, 55 had usable MCA data, and 72 had usable superior sagittal sinus data. This resulted in variable sample sizes for our analyses across vessels. See Fig. 1 for a participant flow chart. The MCA, due to its small size and anatomical variants of both bifurcaton and trifurcations [38], had the most unsuccessful segmentation and overall unusable data.
Fig. 1
Participant Flow Chart. Displays a flow chart that shows the number of participants utilized per specific vessel analysis. Differences in sample size were due to failed vessel segmentation, with the MCA being the most impacted owing to its small size and greater susceptibility to anatomic variation. ICA = 92, Basilar = 75, MCA = 55, SSS = 72. ICA = internal carotid artery, MCA= middle cerebral artery, SSS = superior sagittal sinus.
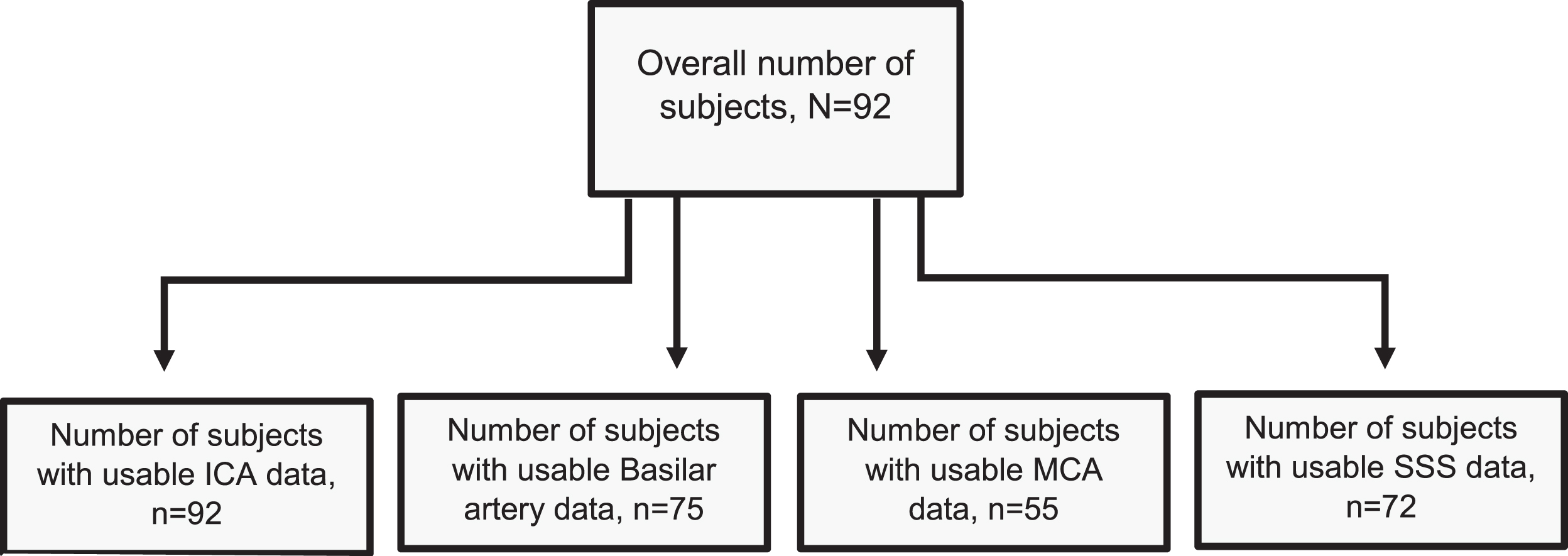
Non-exercise cardiorespiratory fitness measure
Our measure of CRF was based on a previously validated formula: CRF = 18.07 + Sex(2.77) –Age(0.10) –Body Mass Index(0.17) –Resting Heart Rate(0.03) + Self-Reported Physical Activity, with sex coded as female = 0 and male = 1 (28, 39). Standard equation [weight (kg)/height (m)2] was used to calculate body mass index and a GE Dinamap Pro 400 V2 Vital Signs Monitor with a GE Critikon Blood Pressure Cuff was used to determine resting heart rate. Self-reported physical activity was based on the moderate intensity physical activity question from the Women’s Health Initiative physical activity questionnaire [40]. This question inquires into the frequency (range from none to ≥5 days/week) and duration (range from <30 minutes to ≥1 hour/session) of engagement in moderate, “not exhausting”, physical activity (e.g. calisthenics, easy swimming) per week. Following established protocol [40], frequency and duration were multiplied to create a “minutes/week” measure of moderate-intensity physical activity. This measure was then dichotomized to indicate whether (“1”) or not (“0”) an individual obtained 150 minutes of moderate physical activity per week, given national guidelines for physical activity among older adults [28]. This non-exercise measure of CRF has been previously shown to demonstrate good construct validity when assessed against the gold-standard measure of CRF (i.e., VO2peak) [23, 28].
Vascular risk factors
10-year atherosclerotic cardiovascular disease (ASCVD) risk was computed using the American College of Cardiology’s ASCVD Risk Estimator Plus [41]. The ASCVD Risk Estimator Plus online calculator uses the following information: age, race, sex, systolic blood pressure, diastolic blood pressure, cholesterol (total, high density lipoprotein, low density lipoprotein), diabetes status (yes/no), smoking status (current former, never), use of anti-hypertension medication (yes/no), use of statin (yes/no), and use of aspirin therapy (yes/no) to compute an individual’s 10-year ASCVD risk. Hypertension was considered present if there was either a prior diagnosis of hypertension or treatment with anti-hypertensive therapy [42].
Statistical analysis
To determine the relative influence of CRF on PI, we fitted a series of linear regression models (one per vessel) adjusted for age, sex, APOE4 status, and ASCVD risk score. Additionally, a mediation analysis was performed to determine if ASCVD risk score mediated the associtation between CRF and PI using the PROCESS macro v3.4 (43). All analyses were conducted using IBM SPSS, version 25.0. Only findings with a 2-tailed p value ≤0.05 were considered significant.
RESULTS
Background characteristics
Table 1 details demographic information of study participants. Participants were predominantly women (71.7%) with a mean age of 65.34±5.95 years. They were slightly overweight with a mean body mass index of 28.53±5.76 kg/m2 and reported engaging in an average of 60.33±79.78 minutes of moderate intensity physical activity per week. Education was high in our sample (mean = 16.57±2.46 years) and participants’ scores on the MMSE (29.59±0.74) corroborate the cognitive normalcy status of the cohort.
Table 1
Participant Characteristics
Characteristic | Value* |
Demographics | |
Age | 65.34 (5.95) |
Female, % | 71.7 |
Years of education | 16.57 (2.46) |
Non-Hispanic White, % | 95.7 |
Family history positive, % | 69.6 |
APOE4, % | 34.8 |
CRF Estimate Components | |
Amount of moderate physical activity (min/week) | 60.33 (79.78) |
Body mass index (kg/m2) | 28.53 (5.76) |
Resting heart rate (beats/min) | 64.83 (10.13) |
Cognitive and Mood Measures | |
Mini Mental State Exam | 29.59 (0.74) |
CES-D | 6.85 (6.72) |
Vascular Risk Indices | |
Total cholesterol (mg/dL) | 204.83 (40.24) |
HDL cholesterol (mg/dL) | 64.43 (17.56) |
LDL cholesterol (mg/dL) | 119.15 (35.92) |
Glucose (mg/dL) | 96.11 (12.69) |
Insulin (μU/mL) | 10.50 (7.13) |
Systolic blood pressure (mmHg) | 126.8 (17.98) |
Diastolic blood pressure (mmHg) | 74.75 (8.93) |
Hypertension, % | 34.8 |
Diabetes, % | 8.7 |
Smoker (ever), % | 8.7 |
10-year ASCVD risk, % | 11.77 (9.16) |
*All values are mean (s.e.) unless otherwise indicated; APOE4 = apolipoprotein E4; CRF = cardiorespiratory fitness; CES-D = Center for Epidemiologic Studies - Depression Scale; HDL = high-density lipoprotein; LDL = low-density lipoprotein; ASCVD = atherosclerotic cardiovascular disease.
Cardiorespiratory fitness and vessel pulsatility
Table 2 details the results of the regression models that examined the relationship between CRF and vessel PI. There was a significant inverse association between CRF and mean PI in both the inferior (β(SE) = –0.048(0.015), p = 0.001) and superior (β(SE) = –0.034(0.016), p = 0.035) ICAs. There was also a significant inverse association between CRF and PI in the basilar artery (β(SE) = –0.054(0.026), p = 0.040). No other cerebral vessels revealed significant associations (p≥0.228). Figure 2 presents these findings graphically.
Table 2
Association between Cardiorespiratory Fitness and Vessel Pulsatility Index
Vessel | β(SE) | Standardized β | p-value |
Inferior ICA | |||
CRF | –0.048 (0.015) | –0.468 | 0.001 |
Age | 0.001 (0.004) | 0.030 | 0.831 |
Sex | 0.136 (0.065) | 0.322 | 0.038 |
APOE4 status | 0.020 (0.036) | 0.049 | 0.585 |
10-Year ASCVD Risk (%) | 0.006 (0.003) | 0.306 | 0.050 |
Superior ICA | |||
CRF | –0.034 (0.016) | –0.307 | 0.035 |
Age | 0.001 (0.005) | 0.038 | 0.787 |
Sex | 0.045 (0.070) | 0.098 | 0.528 |
APOE4 status | 0.031 (0.039) | 0.071 | 0.434 |
10-Year ASCVD Risk (%) | 0.009 (0.004) | 0.411 | 0.010 |
Basilar Artery | |||
CRF | –0.054 (0.026) | –0.344 | 0.040 |
Age | 0.012 (0.008) | 0.237 | 0.172 |
Sex | 0.067 (0.116) | 0.104 | 0.562 |
APOE4 status | 0.041 (0.069) | 0.067 | 0.559 |
10-Year ASCVD Risk (%) | 0.000 (0.006) | 0.003 | 0.989 |
MCA | |||
CRF | –0.027 (0.022) | –0.237 | 0.228 |
Age | 0.006 (0.007) | 0.168 | 0.443 |
Sex | 0.002 (0.095) | 0.004 | 0.984 |
APOE4 status | 0.029 (0.058) | 0.064 | 0.616 |
10-Year ASCVD Risk (%) | 0.004 (0.005) | 0.209 | 0.376 |
SS Sinus | |||
CRF | –0.005 (0.019) | –0.045 | 0.789 |
Age | 0.009 (0.006) | 0.257 | 0.147 |
Sex | –0.086 (0.088) | –0.171 | 0.334 |
APOE4 status | –0.034 (0.047) | –0.078 | 0.474 |
10-Year ASCVD Risk (%) | 0.005 (0.004) | 0.215 | 0.268 |
ICA = internal carotid artery; CRF = cardiorespiratory fitness; APOE4 = apolipoprotein E4; ASCVD = atherosclerotic cardiovascular disease; MCA = middle cerebral artery; SS Sinus = superior sagittal sinus.
Fig. 2
Regression Analysis of CRF vs Pulsatility Index. Displays the inverse association between CRF and PI in the inferior ICA (A), superior ICA (B), and basilar artery (C). Age, gender, APOE4, and ASCVD risk scores have been factored into these figures. CRF = cardiorespiratory fitness, PI = pulsatility index, ICA = internal carotid artery, APOE4 = apolipoprotein E4, ASCVD = atherosclerotic cardiovascular disease.
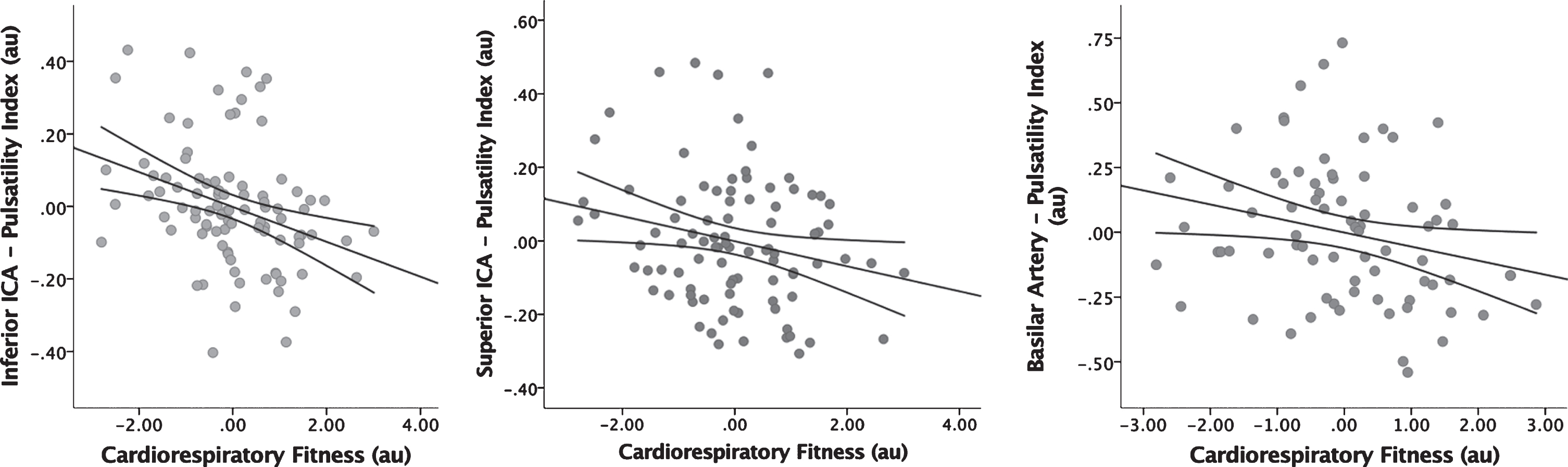
Of the covariates, ASCVD was significantly associated with PI in the inferior (β(SE) = 0.006(0.003), p = 0.050) and superior (β(SE) = 0.009(0.004), p =0.010) ICAs and sex was significantly associated with PI in the inferior ICA (β(SE) = 0.136(0.065), p = 0.038), with women having lower PI. No other covariate exchibited significant associations with PI in any vessel.
Cardiorespiratory fitness, vessel pulsatility, and ASCVD risk score
Given the significant relationship between ASCVD Risk Score and PI in the inferior and superior ICAs, mediation analyses were performed to determine to what extent the relationship between CRF and PI in those vessels is explained by ASCVD (Fig. 3). The mediation model detected a significant association between CRF and ASCVD Risk Score (p = 0.004) as well as confirmed the already observed significant association between ASCVD Risk Score and PI in the inferior (p = 0.050) and superior (p = 0.010) ICAs. The total effect of CRF on inferior ICA PI (p = 0.0001) and the total effect of CRF on superior ICA PI (p = 0.004) were also determined to be significant. However, when ASCVD Risk Score was taken into consideration, the direct effect of CRF on PI in both the inferior and superior ICAs became attenuated by 15.2% and 26.9%, respectively (Fig. 3). These findings are consistent with partial mediation, suggesting that although ASCVD Risk Score plays a role in the association between CRF and PI, other factors are also contributing to that relationship.
Fig. 3
Mediation Analysis of CRF, Vessel PI, and ASCVD Risk Score. Shows ASCVD Risk Score partially mediated the association between CRF and vessel PI in both the inferior (A) and superior ICA (B). Standard coefficients are presented along with standard errors for each path of the mediation model. Note that c represents the total effect and c’ represents the direct effect of CRF on PI. * indicates significance at p < 0.05 and ** indicates significance at p < 0.01. ASCVD = atherosclerotic cardiovascular disease, CRF = cardiorespiratory fitness, PI = pulsatility index, ICA = internal carotid artery.
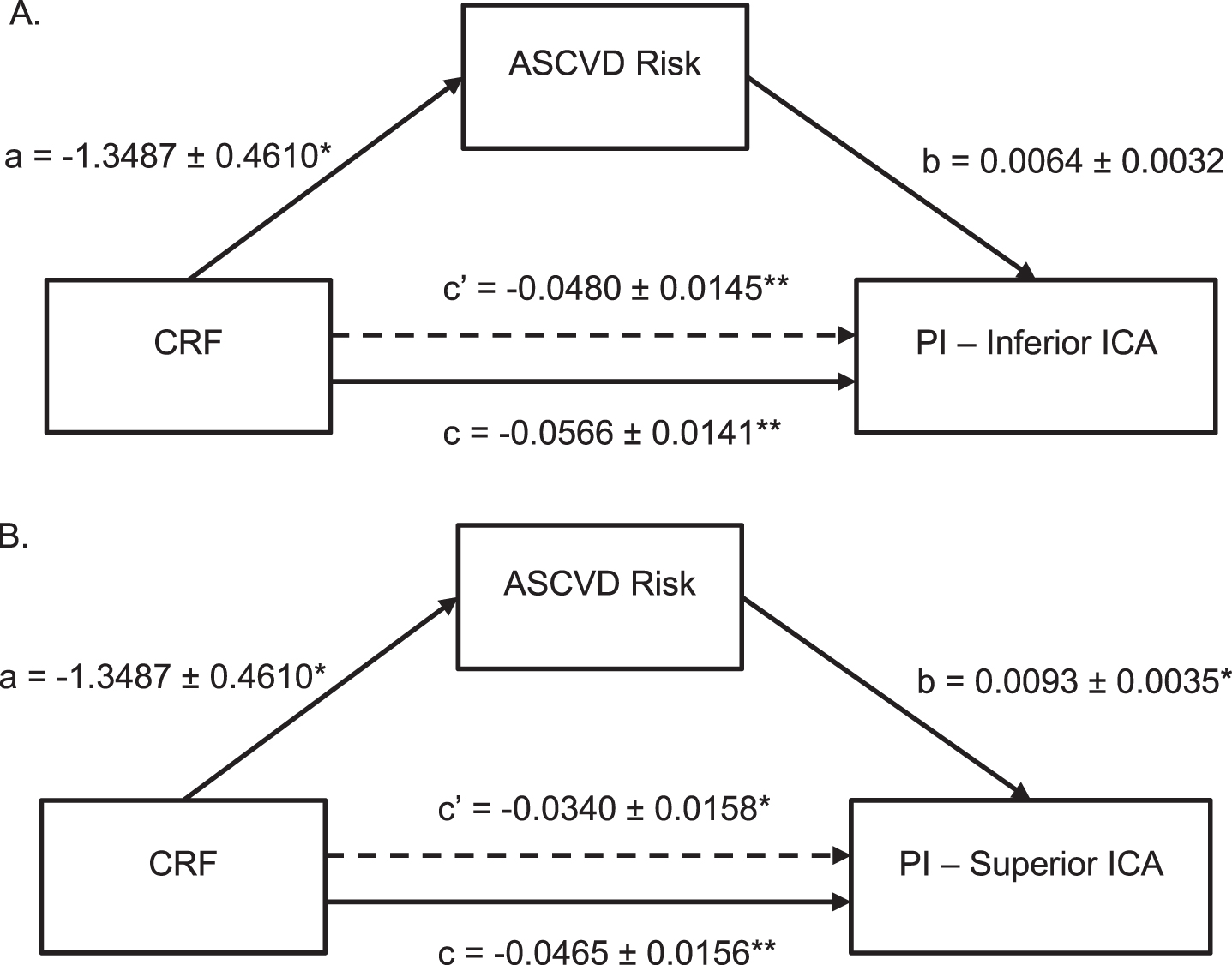
DISCUSSION
The present study revealed that, in a late-middle aged cohort enriched with risk for AD, higher CRF was associated with decreased PI in large cerebral vessels including the inferior and superior ICAs, and the basilar artery. Furthermore, ASCVD Risk Score seemed to partially mediate this relationship between CRF and PI. These data suggest that aerobic fitness might be protective of cerebrovascular health and, since vascular conditions are often co-morbid with AD, the findings suggest that fitness might protect against the manifestation of the dementia syndrome of AD.
Prior studies have shown that CRF influences vascular profiles. There is evidence that an aerobically-active lifestyle attenuates age-related declines in arterial compliance or vessel stiffness [44–50]. Research has shown that individuals with lower CRF exhibit significantly worse arterial stiffening when compared to individuals with higher CRF [44]. One study even reported that regular physical activity could completely abolish age-related arterial stiffening [50]. A difference between these studies and ours is that we focused on PI rather than arterial stiffness. However, both constructs are linked because elevated arterial stiffening is considered to underlie increased cerebral PI [51]. As arteries stiffen, they lose their ability to cushion pulsatile blood flow, constricting the vessel, thus leading to an elevated PI [52]. Therefore, the individuals with greater CRF in our study may have had reduced PI because their engagement in habitual physical activity mitigated arterial stiffening, which in turn, led to lower (decreased) PI. However, future research is needed to confirm this hypothesis. Along those lines, we observed with great interest that our ASCVD Risk Score was significantly associated with both CRF and PI in the inferior and superior ICA. Further, that ASCVD partially mediated the relationship between CRF and PI in those vessels.
The findings of our study are important because a compromised cerebral vasculature has been associated with brain health, cognitive performance, and AD [20, 53]. Decreased mean blood flow and increased pulsatility have been associated with greater brain atrophy [53] and impaired circulation in the cerebral microvasculature [13]. Furthermore, decreased mean blood flow in the ICA and MCA corresponded to worse executive functioning performance in persons with mild cognitive impairment [20]. Previous research comparing the cerebrovasculature of individuals with dementia to healthy controls found that PI of the intracranial arteries is increased in both vascular dementia [13, 54] and AD patients [19, 55, 56]. These studies proposed that the intracranial arterial walls in persons with AD are more rigid, resulting in increased flow resistance and overall decreased arterial compliance [55]. However, the cause of this rigidity is unclear, which is why our study sought to understand whether a vascular risk factor like CRF may affect the cerebrovasculature in individuals enriched with risk for AD.
Previously, vascular aging has been effectively attenuated and treated through habitual physical activity [57, 58]. Furthermore, a growing body of work has begun to examine how lifestyle factors, like physical activity, may help mitigate age-related declines in cerebrovascular integrity [57, 59–61]. In one study, individuals with higher estimated CRF values had increased cerebral blood flow, as quantified by arterial spin labeling, which fully mediated the effect of aging on cerebral perfusion [59]. Another study found that endurance-trained individuals, in comparison to their sedentary counterparts, had MCAs that were “10 years younger” and had higher blood flow velocity, possibly explaining why those who exercise regularly have overall lower risks of cerebrovascular disease [57]. This previous research has focused primarily on the relationship of mean blood flow with exercise. Our study is unique in that it examined the use of PI to describe distal vessel resistance and its relationship to CRF.
The current study uses PC VIPR, a unique 4D flow imaging sequence, to examine how CRF, an index of habitual physical activity, is associated with PI measurements in the large cerebral arteries. We examined a comparatively younger cohort of participants than previous studies [53] and our sample did not contain any individuals with diagnoses of mild cognitive impairment or AD dementia. By examining younger individuals who are not cognitively impaired AD, but at risk for AD, our findings provide support for preventative interventions for AD focused on physical activity. Additionally, our study adds to the growing pool of research conducted on vessel pulsatility and furthers the field by examining how a lifestyle factor like CRF may play a protective role against alterations in the PI of the large cerebral arteries. Because higher PI and increased vessel stiffness have been reported in persons with AD, this suggests that higher CRF may promote vessel compliance and, perhaps by so doing, protect against cerebrovascular changes related to AD clinical syndrome.
A key limitation of our study is that we had some variation in sample sizes across the analyses due to variable levels of success with properly segmenting the cerebral vessels. This might have influenced the comparative statistical significance of the analyses. Another potential limitation is that our sample was predominantly Caucasian, female, and well educated. This lack of demographic diversity potentially limits the generalizability of our findings. Additionally, this study had a cross-sectional design which limits the ability to determine causality in CRF’s association with cerebrovascular pulsatility. Future longitudinal (or interventional) studies would be needed to determine the influence of CRF on prospective brain vascular changes. The self-report measure used for determining CRF is vulnerable to varying forms of biases. Objective measures of CRF, like graded exercise testing, might help circumvent such biases and, therefore, help sharpen estimates of the relationship between fitness and vessel pulsatility. Lastly, the ability to examine additional cerebral vessels (e.g., ACA, PCA) would enable a more comprehsnsive assessment of the effect of CRF on cerebral heamodynamics.
Overall, our study demonstrated that higher CRF is related to lower PI in a late-middle aged cohort enriched with risk for AD. These findings suggest that participation in habitual physical activity could provide protection for cerebrovascular health and thereby confer decreased risk for the evolution of the clinical syndrome of AD. Longitudinal studies would be needed to fully elucidate this hypothesis.
CONFLICT OF INTEREST
C Mitchell: Davies Publishing Inc., authorship textbook. Elsevier, Wolters-Kluwer, author textbook chapters, royalties. Contracted research grants from W.L. Gore & Associates to UW Madison. SCJ has served as an advisor to Roche Diagnostics. No other authors have any conflict of interest to report.
STUDY FUNDING
This work was supported by National Institute on Aging grants K23 AG045957 (OCO), R21 AG051858 (OCO), R01 AG062167 (OCO), R01 AG037639 (BBB), R01 AG027161 (SCJ), R01 AG021155 (SCJ), and P50 AG033514 (SA).
ACKNOWLEDGMENTS
We would like to thank WRAP participants and the WRAP staff for their contributions to the study. Without their participation and efforts, this research would not have been possible.
REFERENCES
[1] | Lane CA , Hardy J , Schott JM . Alzheimer’s disease. Eur J Neurol. (2018) ;25: (1):59–70. |
[2] | Braak H , Braak E . Neuropathological stageing of Alzheimer-related changes. Acta Neuropathol. (1991) ;82: (4):239–59. |
[3] | Viswanathan A , Rocca WA , Tzourio C . Vascular risk factors and dementia: how to move forward? Neurology. (2009) ;72: (4):368–74. |
[4] | Roman GC , Tatemichi TK , Erkinjuntti T , Cummings JL , Masdeu JC , Garcia JH , et al. Vascular dementia: diagnostic criteria for research studies. Report of the NINDS-AIREN International Workshop. Neurology. (1993) ;43: (2):250–60. |
[5] | Ott A , Slooter AJ , Hofman A , van Harskamp F , Witteman JC , Van Broeckhoven C , et al. Smoking and risk of dementia and Alzheimer’s disease in a population-based cohort study: the Rotterdam Study. Lancet. (1998) ;351: (9119):1840–3. |
[6] | Mielke MM , Rosenberg PB , Tschanz J , Cook L , Corcoran C , Hayden KM , et al. Vascular factors predict rate of progression in Alzheimer disease. Neurology. (2007) ;69: (19):1850–8. |
[7] | Sperling RA , Aisen PS , Beckett LA , Bennett DA , Craft S , Fagan AM , et al. Toward defining the preclinical stages of Alzheimer’s disease: recommendations from the National Institute on Aging-Alzheimer’s Association workgroups on diagnostic guidelines for Alzheimer’s disease. Alzheimers Dement. (2011) ;7: (3):280–92. |
[8] | Arvanitakis Z , Capuano AW , Leurgans SE , Bennett DA , Schneider JA . Relation of cerebral vessel disease to Alzheimer’s disease dementia and cognitive function in elderly people: a cross-sectional study. Lancet Neurol. (2016) ;15: (9):934–43. |
[9] | Vemuri P , Knopman DS . The role of cerebrovascular disease when there is concomitant Alzheimer disease. Biochim Biophys Acta. (2016) ;1862: (5):952–6. |
[10] | Penotti M , Farina M , Castiglioni E , Gaffuri B , Barletta L , Gabrielli L , et al. Alteration in the pulsatility index values of the internal carotid and middle cerebral arteries after suspension of postmenopausal hormone replacement therapy: a randomized crossover study. Am J Obstet Gynecol. (1996) ;175: (3 Pt 1):606–11. |
[11] | Kidwell CS , el-Saden S , Livshits Z , Martin NA , Glenn TC , Saver JL . Transcranial Doppler pulsatility indices as a measure of diffuse small-vessel disease. J Neuroimaging. (2001) ;11: (3):229–35. |
[12] | Carroll DJBD . Pulsatility Index (ultrasound). Radiopaedia. 2005. |
[13] | Vicenzini E , Ricciardi MC , Altieri M , Puccinelli F , Bonaffini N , Di Piero V , et al. Cerebrovascular reactivity in degenerative and vascular dementia: a transcranial Doppler study. Eur Neurol. (2007) ;58: (2):84–9. |
[14] | Stefani A , Sancesario G , Pierantozzi M , Leone G , Galati S , Hainsworth AH , et al. CSF biomarkers, impairment of cerebral hemodynamics and degree of cognitive decline in Alzheimer’s and mixed dementia. J Neurol Sci. (2009) ;283: (1-2):109–15. |
[15] | Sattel H , Forstl H , Biedert S . Senile dementia of Alzheimer type and multi-infarct dementia investigated by transcranial Doppler sonography. Dementia. (1996) ;7: (1):41–6. |
[16] | Peters DC , Korosec FR , Grist TM , Block WF , Holden JE , Vigen KK , et al. Undersampled projection reconstruction applied to MR angiography. Magn Reson Med. (2000) ;43: (1):91–101. |
[17] | Chang W , Landgraf B , Johnson KM , Kecskemeti S , Wu Y , Velikina J , et al. Velocity measurements in the middle cerebral arteries of healthy volunteers using 3D radial phase-contrast HYPRFlow: comparison with transcranial Doppler sonography and 2D phase-contrast MR imaging. AJNR Am J Neuroradiol. (2011) ;32: (1):54–9. |
[18] | Wahlin A , Ambarki K , Birgander R , Wieben O , Johnson KM , Malm J , et al. Measuring pulsatile flow in cerebral arteries using 4D phase-contrast MR imaging. AJNR Am J Neuroradiol. (2013) ;34: (9):1740–5. |
[19] | Rivera-Rivera LA , Turski P , Johnson KM , Hoffman C , Berman SE , Kilgas P , et al. 4D flow MRI for intracranial hemodynamics assessment in Alzheimer’s disease. J Cereb Blood Flow Metab. (2016) ;36: (10):1718–30. |
[20] | Berman SE , Clark LR , Rivera-Rivera LA , Norton D , Racine AM , Rowley HA , et al. Intracranial Arterial 4D Flow in Individuals with Mild Cognitive Impairment is Associated with Cognitive Performance and Amyloid Positivity. J Alzheimers Dis. (2017) ;60: (1):243–52. |
[21] | Ford ES , Caspersen CJ . Sedentary behaviour and cardiovascular disease: a review of prospective studies. Int J Epidemiol. (2012) ;41: (5):1338–53. |
[22] | American College of Sports M. ACSM’s guidelines for exercise testing and prescription (9th ed.) Philadelphia: Wolters Kluwer/Lippincott Williams & Wilkins Health; 2014. |
[23] | Mailey EL , White SM , Wojcicki TR , Szabo AN , Kramer AF , McAuley E . Construct validation of a non-exercise measure of cardiorespiratory fitness in older adults. BMC Public Health. (2010) ;10: :59. |
[24] | Colcombe SJ , Erickson KI , Raz N , Webb AG , Cohen NJ , McAuley E , et al. Aerobic fitness reduces brain tissue loss in aging humans. J Gerontol A Biol Sci Med Sci. (2003) ;58: (2):176–80. |
[25] | Colcombe SJ , Kramer AF , Erickson KI , Scalf P , McAuley E , Cohen NJ , et al. Cardiovascular fitness, cortical plasticity, and aging. Proc Natl Acad Sci U S A. (2004) ;101: (9):3316–21. |
[26] | Gordon BA , Rykhlevskaia EI , Brumback CR , Lee Y , Elavsky S , Konopack JF , et al. Neuroanatomical correlates of aging, cardiopulmonary fitness level, and education. Psychophysiology. (2008) ;45: (5):825–38. |
[27] | Johnson NF , Kim C , Clasey JL , Bailey A , Gold BT . Cardiorespiratory fitness is positively correlated with cerebral white matter integrity in healthy seniors. Neuroimage. (2012) ;59: (2):1514–23. |
[28] | Boots EA , Schultz SA , Oh JM , Larson J , Edwards D , Cook D , et al. Cardiorespiratory fitness is associated with brain structure, cognition, and mood in a middle-aged cohort at risk for Alzheimer’s disease. Brain Imaging Behav. (2015) ;9: (3):639–49. |
[29] | Defina LF , Willis BL , Radford NB , Gao A , Leonard D , Haskell WL , et al. The association between midlife cardiorespiratory fitness levels and later-life dementia: a cohort study. Ann Intern Med. (2013) ;158: (3):162–8. |
[30] | Liu R , Sui X , Laditka JN , Church TS , Colabianchi N , Hussey J , et al. Cardiorespiratory fitness as a predictor of dementia mortality in men and women. Med Sci Sports Exerc. (2012) ;44: (2):253–9. |
[31] | Vidoni ED , Honea RA , Billinger SA , Swerdlow RH , Burns JM . Cardiorespiratory fitness is associated with atrophy in Alzheimer’s and aging over 2 years. Neurobiol Aging. (2012) ;33: (8):1624–32. |
[32] | Johnson SC , Koscik RL , Jonaitis EM , Clark LR , Mueller KD , Berman SE , et al. The Wisconsin Registry for Alzheimer’s Prevention: A review of findings and current directions. Alzheimers Dement (Amst). (2018) ;10: :130–42. |
[33] | Okonkwo OC , Schultz SA , Oh JM , Larson J , Edwards D , Cook D , et al. Physical activity attenuates age-related biomarker alterations in preclinical AD. Neurology. (2014) ;83: (19):1753–60. |
[34] | Liu J , Redmond MJ , Brodsky EK , Alexander AL , Lu A , Thornton FJ , et al. Generation and visualization of four-dimensional MR angiography data using an undersampled 3-D projection trajectory. IEEE Trans Med Imaging. (2006) ;25: (2):148–57. |
[35] | Schrauben E , Wahlin A , Ambarki K , Spaak E , Malm J , Wieben O , et al. Fast 4D flow MRI intracranial segmentation and quantification in tortuous arteries. J Magn Reson Imaging. (2015) ;42: (5):1458–64. |
[36] | Kálmán Palágyi EB , KubaCsongor Halmai Attila , Erdőhelyi Balázs , Sorantin Erich , Hausegger Klaus . A Sequential 3D Thinning Algorithm and Its Medical Applications. Information Processing in Medical Imaging. (2080) ;2: :409–15. |
[37] | Katsanos AH , Kosmidou M , Kyritsis AP , Giannopoulos S . Is vertebral artery hypoplasia a predisposing factor for posterior circulation cerebral ischemic events? A comprehensive review. Eur Neurol. (2013) ;70: (1-2):78–83. |
[38] | Navarro-Orozco D , Sanchez-Manso JC . Neuroanatomy, Middle Cerebral Artery. StatPearls. Treasure Island (FL)2020. |
[39] | Jurca R , Jackson AS , LaMonte MJ , Morrow JR Jr , Blair SN , Wareham NJ , et al. Assessing cardiorespiratory fitness without performing exercise testing. Am J Prev Med. (2005) ;29: (3):185–93. |
[40] | McTiernan A , Kooperberg C , White E , Wilcox S , Coates R , Adams-Campbell LL , et al. Recreational physical activity and the risk of breast cancer in postmenopausal women: the Women’s Health Initiative Cohort Study. JAMA. (2003) ;290: (10):1331–6. |
[41] | Cardiology ACo. ASCVD Risk Estimator Plus 2018 [updated November 2018. Available from: https://tools.acc.org/ascvd-risk-estimator-plus/#!/calculate/estimate/ |
[42] | Blaha MJ , Whelton SP , Al Rifai M , Dardari ZA , Shaw LJ , Al-Mallah MH , et al. Rationale and design of the coronary artery calcium consortium: A multicenter cohort study. J Cardiovasc Comput Tomogr. (2017) ;11: (1):54–61. |
[43] | Hayes AF . Introduction to Mediation, Moderation, and Conditional Process Analysis Second Edition A Regression-Based Approach. 2017. |
[44] | Gando Y , Murakami H , Kawakami R , Yamamoto K , Kawano H , Tanaka N , et al. Cardiorespiratory Fitness Suppresses Age-Related Arterial Stiffening in Healthy Adults: A 2-Year Longitudinal Observational Study. J Clin Hypertens (Greenwich). (2016) ;18: (4):292–8. |
[45] | Tanaka H , DeSouza CA , Seals DR . Absence of age-related increase in central arterial stiffness in physically active women. Arterioscler Thromb Vasc Biol. (1998) ;18: (1):127–32. |
[46] | Kawano H , Yamamoto K , Gando Y , Tanimoto M , Murakami H , Ohmori Y , et al. Lack of age-related increase in carotid artery wall viscosity in cardiorespiratory fit men. J Hypertens. (2013) ;31: (12):2370–9. |
[47] | Montalban-Mendez C , Soriano-Maldonado A , Vargas-Hitos JA , Saez-Uran LM , Rosales-Castillo A , Morillas-de-Laguno P , et al. Cardiorespiratory fitness and age-related arterial stiffness in women with systemic lupus erythematosus. Eur J Clin Invest. (2018) ;48: (3). |
[48] | Ferreira I , Twisk JW , Stehouwer CD , van Mechelen W , Kemper HC . Longitudinal changes in. VO2max: associations with carotid IMT and arterial stiffness. Med Sci Sports Exerc. (2003) ;35: (10):1670–8. |
[49] | Quan HL , Blizzard CL , Sharman JE , Magnussen CG , Dwyer T , Raitakari O , et al. Resting heart rate and the association of physical fitness with carotid artery stiffness. Am J Hypertens. (2014) ;27: (1):65–71. |
[50] | Boreham CA , Ferreira I , Twisk JW , Gallagher AM , Savage MJ , Murray LJ . Cardiorespiratory fitness, physical activity, and arterial stiffness: the Northern Ireland Young Hearts Project. Hypertension. (2004) ;44: (5):721–6. |
[51] | Heckmann JG , Hilz MJ , Muck-Weymann M , Neundorfer B . Transcranial doppler sonography-ergometer test for the non-invasive assessment of cerebrovascular autoregulation in humans. J Neurol Sci. (2000) ;177: (1):41–7. |
[52] | Adji A , O’Rourke MF , Namasivayam M . Arterial stiffness, its assessment, prognostic value, and implications for treatment. Am J Hypertens. (2011) ;24: (1):5–17. |
[53] | Berman SE , Rivera-Rivera LA , Clark LR , Racine AM , Keevil JG , Bratzke LC , et al. Intracranial Arterial 4D-Flow is Associated with Metrics of Brain Health and Alzheimer’s Disease. Alzheimers Dement (Amst). (2015) ;1: (4):420–8. |
[54] | Provinciali L , Minciotti P , Ceravolo G , Angeleri F , Sanguinetti CM . Transcranial Doppler sonography as a diagnostic tool in vascular dementia. Eur Neurol. (1990) ;30: (2):98–103. |
[55] | Roher AE , Garami Z , Tyas SL , Maarouf CL , Kokjohn TA , Belohlavek M , et al. Transcranial doppler ultrasound blood flow velocity and pulsatility index as systemic indicators for Alzheimer’s disease. Alzheimers Dement. (2011) ;7: (4):445–55. |
[56] | Rivera-Rivera LA , Schubert T , Turski P , Johnson KM , Berman SE , Rowley HA , et al. Changes in intracranial venous blood flow and pulsatility in Alzheimer’s disease: A 4D flow MRI study. J Cereb Blood Flow Metab. (2017) ;37: (6):2149–58. |
[57] | Ainslie PN , Cotter JD , George KP , Lucas S , Murrell C , Shave R , et al. Elevation in cerebral blood flow velocity with aerobic fitness throughout healthy human ageing. J Physiol. (2008) ;586: (16):4005–10. |
[58] | Seals DR , Walker AE , Pierce GL , Lesniewski LA . Habitual exercise and vascular ageing. J Physiol. (2009) ;587: (Pt 23):5541–9. |
[59] | Zimmerman B , Sutton BP , Low KA , Fletcher MA , Tan CH , Schneider-Garces N , et al. Cardiorespiratory fitness mediates the effects of aging on cerebral blood flow. Front Aging Neurosci. (2014) ;6: :59. |
[60] | Braz ID , Fluck D , Lip GYH , Lundby C , Fisher JP . Impact of aerobic fitness on cerebral blood flow and cerebral vascular responsiveness to CO2 in young and older men. Scand J Med Sci Sports. (2017) ;27: (6):634–42. |
[61] | Tarumi T , Zhang R . Cerebral blood flow in normal aging adults: cardiovascular determinants, clinical implications, and aerobic fitness. J Neurochem. (2018) ;144: (5):595–608. |