Alcohol and Cocaine Combined Substance Use on Adult Hypothalamic Neural Stem Cells and Neurogenesis
Abstract
Many advancements have been made over the years looking at the individual and combined effects of drugs of abuse on the brain, with one key area of research focusing on the effects on neurogenesis. An integral part of fetal brain development and, later, maintenance in the adult brain, neurogenesis occurs in three main regions: subventricularzone of the lateral ventricles (SVZ), subgranularzone of the dentate gyrus (SGZ), and the tanycyte layer in the hypothalamus (TL). We will review current literature on combined drugs of abuse and their effect on adult neurogenesis. More specifically, this review will focus on the effect of combining cocaine and alcohol. Additionally, the tanycyte layer will be explored in more depth and probed to look at the neurogenic properties of tanycytes and their role in neurogenesis.
Neurogenesis and gliogenesis have been extensively studied since the discovery that new neurons and astrocytes, respectively, can be produced from neuronal stem progenitor cells (NSPCs) in two main regions; the subventricular zone (SVZ) of the lateral ventricles and the subgranular zone (SGZ) of the dentate gyrus of the hippocampus [1–3]. Neurogenesis is integral to brain maintenance and repair in the adult brain [3] and studies, primarily focused on the SGZ, have focused on how loss of these cells can alter behavior and cognition [1, 4–6]. More recently, a new neurogenic niche was discovered in the hypothalamus, lining the third ventricle. This region, known as the tanycyte layer (TL), has unique cells called tanycytes which have been identified as neural stem cells due to their ability to self-renew and differentiate [7, 8]; two key properties identifying NSPCs. In addition to this, we have shown that following insult from drugs of abuse there is a marked decrease in NSPC proliferation and differentiation in all three neurogenic regions [9, 10].
TANYCYTES AS HYPOTHALAMIC NEURAL STEM PROGENITOR CELLS
The tanycyte layer of the hypothalamus is becoming recognized as a new neurogenic area. This area was discovered in 1954 [11], however the NSPC-like properties of these cells have only recently been explored. To further clarify, tanycytes were originally subdivided based on their location, along the third ventricle, and morphology into alpha1, alpha2 and beta1, beta2 [12]. Haan et al., explored a distinct subpopulation of these cells that were fibroblast growth factor 10+ (FGF10+) and revealed from P28-P60 there is an increase in proliferation of these NSPCs [8]. However, the exact subpopulation of cells that are NSPCs is still up for debate [13], as another group postulated that the alpha1 cells were the true NSPC population [7]. Our own studies have also suggested that the alpha1 subpopulation is NSPCs [10]. One crucial difference in these studies was the age at which the animals were studied. Haan et al., investigated the tanycyte layer at early postnatal days while two others investigated NSPCs in adult brain [7, 8, 10]. Multiple groups have also investigated this population of cells and have confirmed NSPCs exist in the hypothalamus [14–19]. Unlike the well-studied SVZ and SGZ, the TL has not undergone extensive characterization as to the rate of cell proliferation or turnover in the adult brain. It has been shown, however, that there is an increased rate of NSPC proliferation during development [8] or following insult in the adult brain [9, 10, 17].
While the SGZ zone has been studied for the effects of neurogenesis and behavior, a limited number of studies have addressed behavioral alterations in regard to the TL. More recently, one group has shown that ablating Sox2+ cells in this region leads to an advanced aging phenotype, as well as learning and memory deficits [19]. By using age-matched controls, Zhang et al. was able to show that animals who had undergone NSPC ablation in the hypothalamus expressed locomotor deficits and trouble with memory recall in a novel object recognition task. Additionally, due to their close connection with the arcuate nucleus, which has been implicated to play a large role in feeding behaviors, several groups have suggested that these hypothalamic NSPCs play a role in energy and nutrient sensing [14, 15, 17, 20]. The cell body of tanycytes sits along the third ventricle where they are directly exposed to the cerebrospinal fluid circulation and also arterial blood circulation [15]. Therefore, these cells are in a unique position to relay information to the hypothalamus, and more specifically, the arcuate nucleus. Zhang et al., also proposed NSPCs in the hypothalamus send information via exosomes, and can have indirect effects on distal neurogenic regions by releasing miRNAs [19]. Additionally, since the cell bodies of tanycytes are found along the ventricle they are directly exposed to neurotoxins. One group showed that injecting mono sodium glutamate (3.5 g/kg) systemically resulted in an upregulation of proliferating cells becoming neurons in the arcuate nucleus [17]. Multiple studies have documented neuronal differentiation of hypothalamic NSPCs [7, 8, 17, 21–23], while other studies have focused primarily on glial differentiation [9, 10]. Further investigation is needed in this area to understand why there may be a preferential switch from neuronal to glial differentiation in models of drug use. Thus it is of increasing importance to look at the effects on the tanycytes following substance use and downstream effects on neurogenesis.
COMBINED DRUG USE BACKGROUND
Drugs of abuse have long been studied for their effects on not only the body’s peripheral systems but also their direct effect on the central nervous system. Both legal and illicit drugs can have drastic effects on the brain and behavior [24–26]. These can include short-term effects such as loss of coordination, confusion, and even mood alterations [27]. While focusing on acute drug use is important, increased risk lies in repeated use of these drugs, in not only establishment of a chronic condition, but also due to the fact that use of these substances frequently leads to the development of addiction [28]. Chronic use of drugs of abuse can have a lasting impact on the brain, affecting not only neural connections and impacting behavior, but also causing a loss of key cells, such as NSPCs [9, 10, 29–31]. While most drugs of abuse are studied independently to uncover specific mechanisms of action, it is of translational importance to study combined drug use, due to the high prevalence of combining one or more substances. Combined substance use has been reported to be more common due to the amelioration of negative side effects from one drug by using another [32]. Combined substance use has been linked to a higher risk of relapse following drug taking cessation and a worse prognosis after clinical treatment [33–35]. However, when two drugs are combined we have to consider not only the effects of each drug independently, but also additive or synergistic effects when two drugs are combined [36–38]. This can be potentially fatal, as the drug’s properties can be altered when another substance is present. An example of this is combining cocaine and ethanol, where ethanol is used to ameliorate the negative or “coming down” effect of cocaine [32, 39], and there is the production of a unique metabolite, cocaethylene [36, 40].
COCAINE AND ETHANOL COMBINED DRUG USE CONSEQUENCES AND METABOLISM
In multiple cross-population based studies, it has been reported that a substantial number, around 90%, of cocaine users simultaneously used ethanol to ameliorate the negative side effects and enhance the psychoactive effects of cocaine [33, 35, 41, 42]. This combination is the third most lethal combination of drugs due to cardiac failure [38]. Additionally, the combined use of cocaine and ethanol can result in enhanced psychiatric disorders such as depression, psychotic episodes, and suicidal/homicidal ideation [43–47]. The individual effects of both cocaine and ethanol have been extensively studied [10, 25, 30, 48]. The mechanism of action for cocaine has been described to be primarily mediated by the dopamine and serotonin systems [49–53]. For ethanol, the main CNS-mediated mechanism of action remains more ambiguous due to its small molecular weight and hydrophilic properties, but research suggests the effects of ethanol to be driven by GABA-A receptor activation and NMDA receptor inactivation [54, 55]. Noticeably, the combination of these two drugs is clinically important due to the production of metabolite, cocaethylene [56]. Cocaethylene is only produced when cocaine and ethanol are consumed simultaneously. This is because ethanol inhibits the canonical hydrolysis of cocaine and, instead, induces carboxylesterase 1 (CES1) which metabolizes cocaine into cocaethylene [57–59]. Cocaethylene, similar to cocaine, inhibits dopamine reuptake; and has been shown to have greater euphoric properties than cocaine due to an additive effect following alcohol and cocaine use [52, 60].
Metabolism of drugs of abuse have been well categorized, with the majority of drug metabolism taking place in the liver. However, it has been previously shown that some key metabolic enzymes related to the breakdown of drugs of abuse are present in the brain [61, 62]. It is unknown whether local metabolism of ethanol and cocaine in the brain is harmful or beneficial.
While aldehyde dehydrogenase 1 (ALDH1) is primarily involved in ethanol metabolism, it plays a role in cocaine metabolism by metabolizing 3,4-dihydroxyphenylaldyhde (DOPAL), which is produced following cocaine administration due to the breakdown of extracellular dopamine [63]. ALDH antagonist, disulfiram, has been used clinically for the treatment of ethanol use disorders for decades [64, 65]. Disulfiram has also been used to treat concurrent use of ethanol and cocaine, and shown some efficacy in reducing consumption [64]. Yao and colleagues have also evaluated aldehyde dehydrogenase 2 (ALDH2) antagonists to decrease cocaine-seeking behavior [63]. Further investigation of ALDH antagonism is needed to assess its direct or indirect effect on NSC survival and differentiation in the context of poly-drug abuse.
Cocaethylene, metabolized by CES1 which is found in low levels in the brain [9, 66], has been studied primarily for the effects on the heart, and has been shown to be ten times more cardio-toxic than cocaine [38, 40, 67]. This is due, in part, to the fact that the half-life of cocaethylene is three times longer than cocaine, so effects are sustained for longer periods of time. Additionally, one study has shown cocaethylene can have negative effects on the brain, specifically a loss in righting reflex following intracerebroventricular (ICV) administration [58]. Further research, specifically into the neurotoxic effects of cocaethylene, have yet to be done. Future directions for investigation also include whether cocaethylene is independently neurotoxic to NSPCs and if the production of cocaethylene can precipitate an addictive phenotype greater than cocaine or ethanol alone.
Our laboratory recently established an animal model to begin studying combined substance use of cocaine and ethanol [9]. More importantly, this animal model allowed for the genetic fate-mapping of endogenous NSPCs in the adult brain. Using a Nestin-Cre/LoxP mouse model [68], NSPCs were able to be temporally-and spatially-tracked despite proliferation or differentiation following tamoxifen induction. This allowed for the study of the systemic effects of chronic administration of combined cocaine and ethanol in a mouse model. The study focused on all three neurogenic regions and also investigated sex differences following poly-drug use. Sex differences have been observed in and across multiple drugs of abuse, and this trend holds true for poly-drug use [39, 69–71]. Females reported higher feelings of euphoria following the combination of cocaine and ethanol [39]. Additionally, females have an increased chance of developing psychiatric symptoms [47]. We showed that indeed, across all three neurogenic regions, females had a greater loss of NSPCs than males [9] (Fig. 1). Additionally, astrogliogenesis, or the production of new astrocytes, was upregulated in females more so than males, however the reason behind this still needs to be explored.
Fig. 1
Neural Stem Progenitor Cell Response to Combined Drug Use in Neurogenic Regions. NSPCs are labeled by green fluorescent protein (GFP), nuclei stained with DAPI showing the representative neurogenic regions. SVZ-subventricular zone, SGZ-subgranular zone, TL–tanycyte layer.
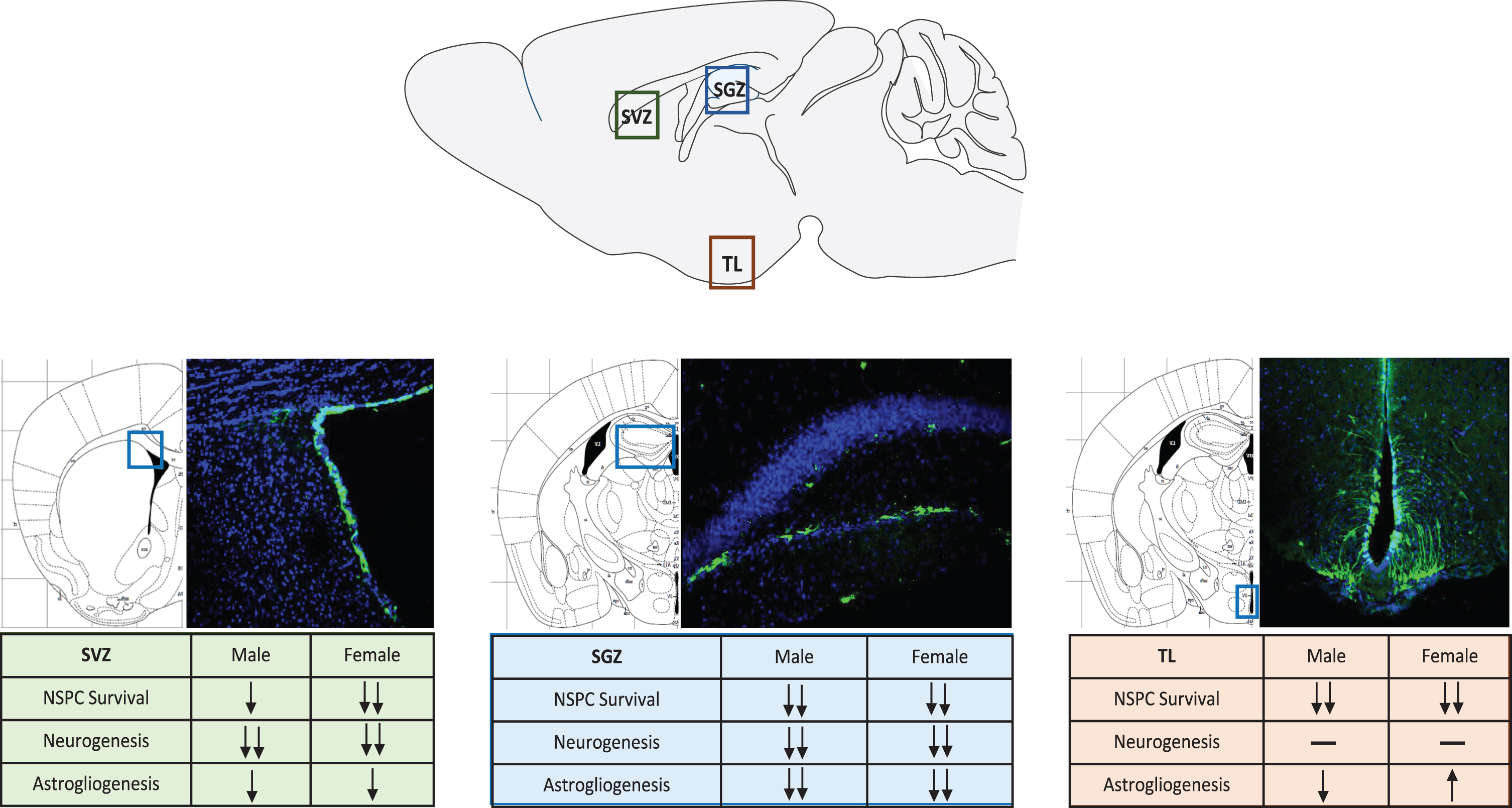
CONCLUSION
Individual use of drugs of abuse have been studied to better understand addictive properties and the impact on behavior and the brain. In addition to this, the effects of alcohol or cocaine on neurogenesis and the negative effects on endogenous NSPC populations has been investigated. With the discovery of the tanycyte layer and identification as a new neurogenicniche, new avenues are now presented, to explore how drugs of abuse can impact NSPCs in this region, directly and indirectly. Additionally, other behaviors, outside of feeding, that can be impacted following loss of NSPCs in the TL need to be investigated. One group showed that loss of these cells, using a viral knockdown approach, can result in an aging-like phenotype as shown by loss of locomotion and learning deficits [19]. Therefore, it would be interesting to see if local administration of these drugs directly to the third ventricle, can produce similar effects.
More recently, combined drug use of cocaine and alcohol in a clinically-translational animal model has been established to further investigate the effects on neurogenesis [9]. Results from the three neurogenic regions showed decreased NSPC survival and differentiation into new neurons, greater than either drug alone. Interestingly, an increase in astrogliogenesis was seen, more often in females than males. Further investigation is needed to explore these results and to probe whether the observed effects are due to additive or synergistic effects of combining alcohol and cocaine. Additionally, further studies need to be conducted to investigate the role of cocaethylene, independently, on the three NSPC populations. Future in vitro studies could also be used by isolating the NSPCs from the three neurogenic regions to more directly investigate the mechanisms behind cell loss and reduced differentiation following drug use.
CONFLICT OF INTERESTS
The authors have no conflict of interest to report.
REFERENCES
[1] | Kempermann G , Song H , Gage FH . Neurogenesis in the Adult Hippocampus. Cold Spring Harb Perspect Biol. (2015) ;7: (9):a018812. |
[2] | Ming GL , Song H . Adult neurogenesis in the mammalian brain: significant answers and significant questions. Neuron. (2011) ;70: (4):687–702. |
[3] | Gage FH . Neurogenesis in the adult brain. J Neurosci. (2002) ;22: (3):612–3. |
[4] | Bannerman DM , et al. Regional dissociations within the hippocampus–memory and anxiety. Neurosci Biobehav Rev. (2004) ;28: (3):273–83. |
[5] | Zhang CL , et al. A role for adult TLX-positive neural stem cells in learning and behaviour. Nature. (2008) ;451: (7181):1004–7. |
[6] | Deng W , Gage FH . The effect of immature adult-born dentate granule cells on hyponeophagial behavior is related to their roles in learning and memory. Front Syst Neurosci. (2015) ;9: :34. |
[7] | Robins SC , et al. α-Tanycytes of the adult hypothalamic third ventricle include distinct populations of FGF-responsive neural progenitors. Nat Commun.. (2013) ;4: :2049. |
[8] | Haan N , et al. Fgf10-expressing tanycytes add new neurons to the appetite/energy-balance regulating centers of the postnatal and adult hypothalamus. J Neurosci. (2013) ;33: (14):6170–80. |
[9] | McGrath EL , et al. Chronic poly-drug administration damages adult mouse brain neural stem cells. Brain Res. 2019:146425. |
[10] | McGrath EL , et al. Spatial and Sex-Dependent Responses of Adult Endogenous Neural Stem Cells to Alcohol Consumption. Stem Cell Reports, 2017. |
[11] | Horstmann E . The fiber glia of selacean brain. Z Zellforsch Mikrosk Anat. (1954) ;39: (6):588–617. |
[12] | Millhouse OE . A Golgi study of third ventricle tanycytes in the adult rodent brain. Z Zellforsch Mikrosk Anat. (1971) ;121: (1):1–13. |
[13] | Schlagal CR , Wu P . Tipsy neural stem cells: chronic effects of alcohol on the brain. Neural Regen Res. (2019) ;14: (1):67–8. |
[14] | Kokoeva MV , Yin H , Flier JS . Neurogenesis in the hypothalamus of adult mice: potential role in energy balance. Science. (2005) ;310: (5748):679–83. |
[15] | Rodríguez EM , et al. Hypothalamic tanycytes: a key component of brain-endocrine interaction. Int Rev Cytol. (2005) ;247: :89–164. |
[16] | Prevot V , et al. The versatile tanycyte: a hypothalamic integrator of reproduction and energy metabolism. Endocr Rev, 2018. |
[17] | Yulyaningsih E , et al. Acute Lesioning and Rapid Repair of Hypothalamic Neurons outside the Blood-Brain Barrier. Cell Rep. (2017) ;19: (11):2257–71. |
[18] | Kokoeva MV , Yin H , Flier JS . Evidence for constitutive neural cell proliferation in the adult murine hypothalamus. J Comp Neurol. (2007) ;505: (2):209–20. |
[19] | Zhang Y , et al. Hypothalamic stem cells control ageing speed partly through exosomal miRNAs. Nature. (2017) ;548: (7665):52–7. |
[20] | Pierce AA , Xu AW . De novo neurogenesis in adult hypothalamus as a compensatory mechanism to regulate energy balance. J Neurosci. (2010) ;30: (2):723–30. |
[21] | Lee DA , Blackshaw S . Functional implications of hypothalamic neurogenesis in the adult mammalian brain. Int J Dev Neurosci. (2012) ;30: (8):615–21. |
[22] | Lee DA , et al. Tanycytes of the hypothalamic median eminence form a diet-responsive neurogenic niche. Nat Neurosci. (2012) ;15: (5):700–2. |
[23] | Zhang Y , et al. Metabolic learning and memory formation by the brain influence systemic metabolic homeostasis. Nat Commun. (2015) ;6: :6704. |
[24] | Korpi ER , et al. Mechanisms of Action and Persistent Neuroplasticity by Drugs of Abuse. Pharmacol Rev. (2015) ;67: (4):872–1004. |
[25] | Crews FT , Nixon K . Alcohol, neural stem cells, and adult neurogenesis. Alcohol Res Health. (2003) ;27: (2):197–204. |
[26] | Kutlu MG , Gould TJ . Effects of drugs of abuse on hippocampal plasticity and hippocampus-dependent learning and memory: contributions to development and maintenance of addiction. Learn Mem. (2016) ;23: (10):515–33. |
[27] | Thompson JP . Acute effects of drugs of abuse. Clin Med (Lond). (2003) ;3: (2):123–6. |
[28] | Weiss F , et al. Compulsive drug-seeking behavior and relapse. Neuroadaptation, stress, and conditioning factors. Ann N Y Acad Sci. (2001) ;937: :1–26. |
[29] | He J , et al. Chronic alcohol exposure reduces hippocampal neurogenesis and dendritic growth of newborn neurons. Eur J Neurosci. (2005) ;21: (10):2711–20. |
[30] | Sudai E , et al. High cocaine dosage decreases neurogenesis in the hippocampus and impairs working memory. Addict Biol. (2011) ;16: (2):251–60. |
[31] | Mandyam CD , Koob GF . The addicted brain craves new neurons: putative role for adult-born progenitors in promoting recovery. Trends Neurosci. (2012) ;35: (4):250–60. |
[32] | Magura S , Rosenblum A . Modulating effect of alcohol use on cocaine use. Addict Behav. (2000) ;25: (1):117–22. |
[33] | Schmitz JM , et al. Treatment outcome of cocaine-alcohol dependent patients. Drug Alcohol Depend. (1997) ;47: (1):55–61. |
[34] | Mengis MM , et al. Alcohol use affects the outcome of treatment for cocaine abuse. Am J Addict. (2002) ;11: (3):219–27. |
[35] | Lyne J , et al. Concurrent cocaine and alcohol use in individuals presenting to an addiction treatment program. Ir J Med Sci. (2010) ;179: (2):233–7. |
[36] | Henning RJ , Wilson LD . Cocaethylene is as cardiotoxic as cocaine but is less toxic than cocaine plus ethanol. Life Sci. (1996) ;59: (8):615–27. |
[37] | Koski A , Ojanperä I , Vuori E . Interaction of alcohol and drugs in fatal poisonings. Hum Exp Toxicol. (2003) ;22: (5):281–7. |
[38] | Wilson LD , et al. Cocaine, ethanol, and cocaethylene cardiotoxity in an animal model of cocaine and ethanol abuse. Acad Emerg Med. (2001) ;8: (3):211–22. |
[39] | Graziani M , Nencini P , Nisticò R . Genders and the concurrent use of cocaine and alcohol: Pharmacological aspects. Pharmacol Res. (2014) ;87: :60–70. |
[40] | Wilson LD , et al. Cocaethylene causes dose-dependent reductions in cardiac function in anesthetized dogs. J Cardiovasc Pharmacol. (1995) ;26: (6):965–73. |
[41] | United States. Department of Health and Human Services, Facing addiction in America : the Surgeon General’s report on alcohol, drugs and health. HHS publication. 2016,W ashington DC: U.S. Department of Health & Human Services. 1 volume (various pagings). |
[42] | Midanik LT , Tam TW , Weisner C . Concurrent and simultaneous drug and alcohol use: results of the 2000 National Alcohol Survey. Drug Alcohol Depend. (2007) ;90: (1):72–80. |
[43] | Salloum IM , et al. Concurrent alcohol and cocaine dependence impact on physical health among psychiatric patients. J Addict Dis. (2004) ;23: (2):71–81. |
[44] | Salloum IM , et al. Disproportionate lethality in psychiatric patients with concurrent alcohol and cocaine abuse. Am J Psychiatry. (1996) ;153: (7):953–5. |
[45] | Goldstein RZ , et al. Severity of neuropsychological impairment in cocaine and alcohol addiction: association with metabolism in the prefrontal cortex. Neuropsychologia. (2004) ;42: (11):1447–58. |
[46] | Garlow SJ , Purselle DC , Heninger M . Cocaine and alcohol use preceding suicide in African American and white adolescents. J Psychiatr Res. (2007) ;41: (6):530–6. |
[47] | Velasquez MM , et al. Psychiatric distress in incarcerated women with recent cocaine and alcohol abuse. Womens Health Issues. (2007) ;17: (4):264–72. |
[48] | Nixon K , Crews FT . Binge ethanol exposure decreases neurogenesis in adult rat hippocampus. J Neurochem. (2002) ;83: (5):1087–93. |
[49] | Bradberry CW , et al. Cocaine and cocaethylene: microdialysis comparison of brain drug levels and effects on dopamine and serotonin. J Neurochem. (1993) ;60: (4):1429–35. |
[50] | Bradberry CW . Dynamics of extracellular dopamine in the acute and chronic actions of cocaine. Neuroscientist. (2002) ;8: (4):315–22. |
[51] | Cunningham KA , et al. The role of serotonin in the actions of psychostimulants: molecular and pharmacological analyses. Behav Brain Res. (1996) ;73: (1-2):93–102. |
[52] | Jatlow P . Cocaethylene: pharmacologic activity and clinical significance. Ther Drug Monit. (1993) ;15: (6):533–6. |
[53] | Ritz MC , Cone EJ , Kuhar MJ . Cocaine inhibition of ligand binding at dopamine, norepinephrine and serotonin transporters: a structure-activity study. Life Sci. (1990) ;46: (9):635–45. |
[54] | Allan AM , Harris RA . Gamma-aminobutyric acid and alcohol actions: neurochemical studies of long sleep and short sleep mice. Life Sci. (1986) ;39: (21):2005–15. |
[55] | Valenzuela CF . Alcohol and Neurotransmitter Interactions. 1997, Alcohol Health & Research World. p. 144-148. |
[56] | McCance-Katz EF , Kosten TR , Jatlow P , Concurrent use of cocaine and alcohol is more potent and potentially more toxic than use of either alone–a multiple-dose study. Biol Psychiatry. (1998) ;44: (4):250–9. |
[57] | Laizure SC , et al. Cocaethylene metabolism and interaction with cocaine and ethanol: role of carboxylesterases. Drug Metab Dispos. (2003) ;31: (1):16–20. |
[58] | Wilson DM , et al. The interaction of dopamine, cocaine, and cocaethylene with ethanol on central nervous system depression in mice. Pharmacol Biochem Behav. (1997) ;57: (1-2):73–80. |
[59] | Landry MJ . An overview of cocaethylene, an alcohol-derived, psychoactive, cocaine metabolite. J Psychoactive Drugs. (1992) ;24: (3):273–6. |
[60] | Hearn WL , et al. Cocaethylene: a unique cocaine metabolite displays high affinity for the dopamine transporter. J Neurochem. (1991) ;56: (2):698–701. |
[61] | Hipólito L , et al. Brain metabolism of ethanol and alcoholism: an update. Curr Drug Metab. (2007) ;8: (7):716–27. |
[62] | Ferguson CS , Tyndale RF . Cytochrome P450 enzymes in the brain: emerging evidence of biological significance. Trends Pharmacol Sci. (2011) ;32: (12):708–14. |
[63] | Yao L , et al. Inhibition of aldehyde dehydrogenase-2 suppresses cocaine seeking by generating THP, a cocaine use-dependent inhibitor of dopamine synthesis. Nat Med. (2010) ;16: (9):1024–8. |
[64] | Carroll KM . Implications of recent research for program quality in cocaine dependence treatment. Subst Use Misuse. (2000) ;35: (12-14):2011–30. |
[65] | Carroll KM , et al. Treatment of cocaine and alcohol dependence with psychotherapy and disulfiram. Addiction. (1998) ;93: (5):713–27. |
[66] | Wang D , et al. Human carboxylesterases: a comprehensive review. Acta Pharm Sin B. (2018) ;8: (5):699–712. |
[67] | Qiu Z , Morgan JP . Differential effects of cocaine and cocaethylene on intracellular Ca2+ and myocardial contraction in cardiac myocytes. Br J Pharmacol. (1993) ;109: (2):293–8. |
[68] | Lagace DC , et al. Dynamic contribution of nestin-expressing stem cells to adult neurogenesis. J Neurosci. (2007) ;27: (46):12623–9. |
[69] | Bobzean SA , DeNobrega AK , Perrotti LI . Sex differences in the neurobiology of drug addiction. Exp Neurol. (2014) ;259: :64–74. |
[70] | Becker JB . Sex differences in addiction. Dialogues Clin Neurosci. (2016) ;18: (4):395–402. |
[71] | Becker JB , Chartoff E . Sex differences in neural mechanisms mediating reward and addiction. Neuropsychopharmacology. (2019) ;44: (1):166–83. |