Evaluating Exercise as a Therapeutic Intervention for Methamphetamine Addiction-Like Behavior1
Abstract
The need for effective treatments for addiction and dependence to the illicit stimulant methamphetamine in primary care settings is increasing, yet no effective medications have been FDA approved to reduce dependence [1]. This is partially attributed to the complex and dynamic neurobiology underlying the various stages of addiction [2]. Therapeutic strategies to treat methamphetamine addiction, particularly the relapse stage of addiction, could revolutionize methamphetamine addiction treatment. In this context, preclinical studies demonstrate that voluntary exercise (sustained physical activity) could be used as an intervention to reduce methamphetamine addiction. Therefore, it appears that methamphetamine disrupts normal functioning in the brain and this disruption is prevented or reduced by engaging in exercise. This review discusses animal models of methamphetamine addiction and sustained physical activity and the interactions between exercise and methamphetamine behaviors. The review highlights how methamphetamine and exercise affect neuronal plasticity and neurotoxicity in the adult mammalian striatum, hippocampus, and prefrontal cortex, and presents the emerging mechanisms of exercise in attenuating intake and in preventing relapse to methamphetamine seeking in preclinical models of methamphetamine addiction.
INTRODUCTION
For centuries, there has been widespread interest to understand the importance of physical activity on the overall quality of life of an individual. The idea that stress and mental states have adverse effects on physical health is well accepted, and conversely, it is suggested that a healthy body will espouse a healthy mind [3]. Thus, an emerging area of interest focuses on the mechanisms by which exercise (sustained physical activity) affects the mammalian brain, particularly on cognitive function. One way to conduct research in this field involves the use animal models to delve more deeply into cortical connectivity and molecular and cellular mechanisms involved in exercise and brain function. This micro-view allows us to see major benefits (and minor pitfalls) of exercise that may otherwise be easily overlooked.
Addiction or substance dependence is a mental disorder that involves the loss of behavioral control over drug taking and drug seeking which involves impulsivity and compulsivity. The impulsive and compulsive features of addiction can be grouped into three stages: [1] binge/prolonged intoxication, [2] withdrawal neutral/negative affect, and [3] preoccupation/anticipation (craving); these stages comprise the cycle of addiction. The limited (often recreational) use of drugs with abuse potential is distinct from the pattern of escalated drug use and the materialization of a chronic drug-dependent state [4]. Furthermore, the last stage of the addiction cycle describes a key element of addiction in humans which defines addiction as a chronic relapsing disorder [2]. Understanding the neurobiology of addiction is critical for identifying therapeutic strategies, and in this regard animal models of addiction have proved to be an immensely valuable tool for parsing out the neurobiological adaptations that contribute to the progression of addiction through these various stages [2, 5, 6].
A recent review detailed the value of exercise as a potential therapeutic strategy for intervention during the various stages of addiction for several drugs of abuse [7]. The therapeutic value of exercise could be due to its natural reinforcing effects and it may contribute to reward interaction, whereby, the presence of two reinforcing agents (e.g. illicit drug and exercise) could devalue the alternate reinforcer [8, 9]. The current review focuses on methamphetamine, a psychostimulant illicit drug with high abuse potential, with the objective to understand where the interaction between methamphetamine and exercise may be beneficial. This review discusses the neurobiological adaptations produced by exercise which contribute to reversing the negative effects of methamphetamine, in addition to exploring the theory of exercise itself eliciting addictive behavior.
METHAMPHETAMINE ADDICTION: IMPACT ON SOCIETY
The burden of methamphetamine abuse is increasing in the United States; available SAMHSA reports show 8% of all drug and alcohol treatment admissions involve methamphetamine and treatment studies report frequent relapses to methamphetamine seeking among those that are trying to quit [10]. Furthermore, methamphetamine abuse takes emotional and financial tolls on society, cutting across ages, races, ethnicities, and genders. It increases mortality, morbidity, and economic costs. Therefore, successfully reducing risk-taking behaviors, such as methamphetamine abuse, can potentially result in large public health gains [11, 12].
According to the recent reports from the National Institutes on Drug abuse (NIDA) and others, there are a few treatment options for methamphetamine addiction [1, 13–15]. For example, the best therapy currently available is the behavioral treatment approach (Matrix model, an outpatient treatment using psychosocial protocols, [16]); however, behavioral therapies are associated with lower beneficial effects on maintaining an individual’s abstinence as well as reducing drug craving elicited by drug-related context and cues [17]. Therefore, NIDA is currently conducting research to seek improved treatment options, including investigating the efficacy of non-traditional and non-pharmaceutical options that may treat methamphetamine addiction.
PHYSICAL FITNESS VIA EXERCISE: IMPACT ON SOCIETY
In the world we live in today, almost everything we need is available at the click of a mouse. As an unfortunate consequence, it has become too easy to avoid physical activity and slip into a sedentary lifestyle, which has been associated with obesity, diabetes mellitus, increased risk of cardiovascular disease, and direct and indirect socioeconomic costs [18–21]. In recent years, the benefits of exercise on health and wellness have become increasingly well known. Exercise has been found to reduce the severity and even reverse the previously stated medical conditions associated with physical inactivity, which in turn reduces the cost of medical needs and other indirect socioeconomic costs [18, 22, 23]. Perhaps more relevant to this review, exercise can improve mental health by offering relief from the symptoms of depression and anxiety, and by improving mood [24–26]; these effects make exercise a valuable tool in disrupting the vicious feed-forward loop between mental illness and substance abuse [27–29].
While regular and regimented physical activity may be therapeutic, clinical cases of exercise eliciting addiction-like behavior have been reported with many sports activities including running, martial arts, weight lifting and body-building, and have been summarized in The Exercise Paradox [30]. The latter study used the Exercise Dependence Scale and the Exercise Addiction Inventory and reported that between 3.4% and 13.4% of a sample of university students were at high risk for “exercise addiction” [30]. Clinical cases have reported loss of control over exercise behavior and compulsivity or ‘obligation’ to perform exercise [30], both of which are hallmarks of addictive disorders [31]. Salience, withdrawal, emotional dysregulation, negative physical and psychosocial consequences, tolerance to the quantity and/or intensity, and relapse to abuse behavior are other phenotypes that support the notion that exercise may be an addictive behavior [30, 32–34]. However, because exercise is considered a sociologically normative behavior, addiction to exercise may be difficult to detect. Thus, partially due to the paucity of peer-reviewed evidence, “exercise addiction” is not yet categorized in Diagnostic and Statistical Manual of Mental Diseases or International Classification of Diseases [31, 35]. Furthermore, excessive preoccupation with exercise has been observed with other disorders, including eating disorders and body dysmorphic disorders [33, 34, 36, 37]. This begs the question whether addiction-like exercise behavior is manifested due to vulnerability of specific personality types, or caused by excessive exposure to exercise. Animal models are more useful at parsing out such causalities.
EXERCISE AS A TREATMENT OPTION FOR METHAMPHETAMINE ADDICTION
In the context of substance abuse disorders, such as methamphetamine addiction, a single pharmacological intervention may not be sufficient or useful throughout the progression of addiction because the underlying neurobiology of addiction is continually changing between the different stages of addiction [2, 38]. Exercise is reported to have negative relationships with the extent, duration and frequency of drug use throughout the stages of initiation, prolonged intoxication, withdrawal, and relapse [7], and thus, has been sought out as a potential non-pharmacological treatment. Moderate exercise in methamphetamine dependent individuals significantly reduced depression symptom scores; in fact, “individuals with the most severe medical, psychiatric and addiction disease burden at baseline showed the most significant improvement in depressive symptoms by study endpoint” [39]. Similarly, group exercise for substance use disorder patients was found to significantly improve the physical health and psychological health domains of quality of life questionnaire; this intervention was beneficial even to patients with the most severe health problems [40]. Since depression and poor mental health are risk factors for relapse to drug seeking [27–29], these studies suggest that exercise is a useful strategy for reducing relapse in methamphetamine dependent individuals. Most importantly, the low cost and ease-of-implementation make exercise therapy all the more lucrative as a potential intervention. Therefore, clinical studies suggest that exercise can be used as a therapeutic intervention that may treat methamphetamine addiction, particularly during the relapse stage of addiction. The rest of the review will focus on the preclinical models of methamphetamine addiction and of exercise eliciting addiction like behavior. Furthermore, we hope to provide a brief overview on the neuroprotective effects of exercise on methamphetamine addiction in the context of inhibition of intake.
ANIMAL MODELS OF ADDICTION TO ILLICIT DRUGS
Drug-taking behavior has been demonstrated in rodent models of intravenous drug self-administration, in which rodents are trained to self-administer drugs by pressing a lever for an intravenous drug infusion in an operant conditioning chamber [41]. As mentioned previously, addiction is distinct from limited or recreational drug use. An increase in drug availability or a history of drug intake has been shown to accelerate the development of dependence in humans [42, 43]. Thus, animal models of addiction should highlight dependence-like behaviors, such as escalation of drug intake over time and withdrawal symptoms when the drug is withheld [41]. In that regard, intravenous methamphetamine self-administration with intermittent (1 h biweekly), limited (1 h daily), or long (extended; >4 h daily) access has significant clinical relevance by illustrating a range of different methamphetamine seeking behaviors in rats [44]. Extended access to methamphetamine produces an escalation of methamphetamine self-administration, suggesting compulsive drug intake and reflecting dependence-like behavior. Therefore, the escalation model may provide a useful approach to understand the neurobiological mechanisms responsible for the transition from limited methamphetamine use to compulsive intake. The escalation model may be particularly suitable for testing the hypothesis that alterations in adult brain plasticity induced by the methamphetamine are partially responsible for addictive behavior [41].
The reinstatement of drug-seeking behavior in rats is widely used as a model of craving that mimics the relapse stage of addiction often observed in the clinic [45], and is used extensively to uncover the key brain regions, brain circuitry, neurotransmitters, and neuromodulators associated with reinstatement behavior. The paradigm extinguishes learned self-administration behavior by explicitly not rewarding (e.g. withholding intravenous methamphetamine infusion) the animal after the correct response is emitted (e.g. a correct lever press) and tests the ability of a priming stimulus to reinstate drug-seeking response [46]. The stimuli can be cues previously paired with drug self-administration (cue priming) or acute noncontingent exposure to the drug (experimenter delivered; i.e., drug priming) or context (spatial location) where the drug was self-administered. The drug seeking reinstatement model has been used to mimic an addict’s drug-response pattern [47], and mimic high rates of relapse [47, 48].
An alternative approach for assessing drug reward involves conditioned place preference (CPP), which determines the Pavlovian associations between rewarding effects of a drug and a contiguously presented stimulus, i.e. the context in which the rewarding effects of the drug are experienced [49, 50]. This conditioning approach is different from operant behavior, as it does not involve the animal ‘working’ for the drug reward (e.g. no lever responses), but rather, it examines the preference for the context following the development of the association [51]. Furthermore, in this model animals receive passive administration of the drug (i.e. experimenter delivered), and are tested (cue/context) in a drug-free state [50, 52]. Although the operant self-administration and CPP models have face validity, the operant model of drug reward and reinstatement is more advantageous. For example, while CPP behavior fails to demonstrate drug dose-dependency, the self-administration model is useful for eliciting distinct drug intake patterns (limited vs. compulsive intake of the drug) to mimic recreational use vs. dependent use, and to model an addict’s drug-response pattern, and high relapse rates. Thus, the intravenous self-administration model of drug exposure appears to be the best suited for studying the neural mechanisms of drug reward and relapse.
ANIMAL MODELS OF SUSTAINED PHYSICAL ACTIVITY
Various animal models of exercise have been utilized which can be broadly classified as either voluntary or as forced exercise [53]. One of the most commonly used exercise paradigms involves the use of running wheels. In a voluntary wheel running paradigm, a wheel is placed in the home cage of the animal and the animal has ad libitum access to the wheel. In contrast, forced wheel running isolates the animal with the wheel and partitions off access to any other area; rotation of the wheel can be controlled remotely (using a computer or other mechanism) to ensure that the animal is forced to run while on the wheel [53]. Another forced exercise animal model is treadmill running, where the animal is forced to run on a motor-driven treadmill for a controlled amount of time [53]. Swimming has also been used as an exercise model where the animal was forced to swim in a tank with access to a platform for resting (optional) without leaving the water [54, 55]. Both voluntary and forced exercise paradigms have proven effective animal models; while voluntary exercise is typically chosen to simulate human choices for physical activity, forced exercise is suggested to more closely resemble human exercise regiments [56]. Environmental enrichment is another technique that provides greater opportunity for physical activity compared to standard housing conditions [53], but is a more complex paradigm with social and cognitive stimulation additionally involved; thus, environmental enrichment will not be discussed in the present review.
Utilizing these models of sustained physical activity, there is emerging evidence from animal studies that exercise is addictive and the behavioral outcomes can be compared with other drugs of abuse [8]. For example, the binge/intoxication phase of addiction is characterized by escalation of drug intake; in voluntary wheel running paradigms, escalation of running activity over days of access has been reported by several groups [57–60]. Such behavior indicates that exercise is reinforcing and can induce increased use over time. Additionally, using the CPP task, rats were reported to prefer the chamber previously paired with running wheels (wheel-paired context) compared to the chamber without wheel-pairing history [57], suggesting that access to running wheel is rewarding. Interestingly, physical exercise has been shown to activate the same mesolimbic reward pathway as illicit drugs; like methamphetamine, exercise can increase extracellular dopamine in the ventral striatum (Fig. 1), thus further identifying the neuronal mechanism underlying the rewarding and reinforcing effects of exercise [57, 61]. Combined, this body of research suggests that exercise is rewarding, and can illicit behaviors that correspond to the binge/intoxication phase ofaddiction.
The negative affect and withdrawal symptoms that are typical to drug dependence have also been observed in humans when experiencing a withdrawal from physical exercise [30]; some of these behavioral and physical aspects can also be found in animal models. For example, in mice that were selectively bred for high wheel running activity, providing access to running wheels and subsequently removing wheels access (withdrawal) lead to reduction of systolic and diastolic blood pressure, and of body temperatures, when compared with inbred mice with continued access to wheels or to control mice [62]. Evidence for the negative affect in animal models of drug addiction include behavioral symptoms such as depression and anxiety [63, 64]. Similar observations were made in exercise-preferring inbred mice that were withdrawn from running wheel activity, in whom behavioral despair or depression, assessed using the forced swim test, was elevated compared to control mice [65]. Taken together, animals that are genetically predisposed for high levels of physical activity and are then not permitted to engage in such activity, showed classical signs of withdrawal similar to those seen in drug dependent animals. However, these effects are not restricted to animals that are selectively bred for high wheel running. Outbred rats that were given free access to running wheels for 30 days and then prevented access to wheels for 24 hours were more likely to ‘relapse’ or binge on running after the wheels were reintroduced [60]. Because drug-addiction is a chronic relapsing disorder, the latter study provides strong evidence that, like drugs of abuse, running wheels can elicit relapse behavior. In conclusion, exercise does elicit addictive behavior, and this effect is enhanced in the genetically vulnerable strain.
CONVERGENCE BETWEEN EXERCISE AND METHAMPHETAMINE ADDICTION IN ANIMAL MODELS
There is an extensive amount of preclinical research supporting exercise as a treatment for drug addiction where exercise has been repeatedly shown to reduce the amount of drug intake during self-administration sessions (for review [7]). With respect to methamphetamine, exercise regimens have reduced self-administration during acquisition [58, 66] and during escalation of intake [58]. Research conducted in our laboratory also shows that access to exercise (voluntary wheel running) during protracted withdrawal from methamphetamine self-administration improved the rate of extinction and reduced both context- and cue-induced reinstatement of methamphetamine seeking [59]. Exercise also inhibits amphetamine reward as evidenced by inhibition of CPP for amphetamine following forced treadmill running [67]. However, withdrawal from access to running wheels was found to increase methamphetamine intake during acquisition compared to rats with continued access to wheels as well as compared to exercise naïve rats [58]. Furthermore, escalation of methamphetamine intake did not differ between exercise-withdrawn rats and exercise-naïve rats [58], suggesting that the protective effect of exercise in methamphetamine addiction may be contingent on continued availability of the running wheel. This lends further credence to the hypothesis that the efficacy of exercise depends on its ability to serve as a strong alternate rewarding and reinforcing agent. The neurobiological bases underlying the effects of exercise in methamphetamine addiction as well as the adaptations underlying the addictive effects of exercise will be explored in the subsequent sections.
NEURAL CIRCUITRY UNDERLYING METHAMPHETAMINE AND EXERCISE ADDICTION
The reward and relapse circuitry in the adult mammalian brain has been delineated based on multiple groundbreaking studies performed in rodent models of acute and chronic reinforcement schedules and reinstatement to drug-seeking behavior [45, 68]. The acute reinforcing actions of stimulants, like methamphetamine, has been attributed to the mesolimbic dopamine pathway that initiates in the ventral tegmental area and terminates in the nucleus accumbens (ventral striatum; the brain reward center); however, it is important to note that for sedative/hypnotics and opiates, such as ethanol and heroin, these regions are not critical for the acute reinforcing effects [2]. Furthermore, there is evidence that neurotransmitters other than dopamine may also play a significant role in the rewarding effects of stimulants. For example, the nucleus accumbens receives inputs from several other brain regions, including the medial prefrontal cortex and hippocampal regions [2], and notably, the neurotransmitter glutamate from these regions regulates dopamine release from the nucleus accumbens and ventral tegmental area [69–71]. These results suggest that illicit drugs dysregulate prefrontocortical and hippocampal neurocircuitry, and thereby, contribute to the enhanced dopamine release from the mesolimbic dopamine pathway, and all of these regions play critical roles in the acute reinforcing effects of stimulant drugs [2].
The key brain regions implicated in the reinstatement of drug-seeking behavior include, but are not limited to, the medial prefrontal cortex, nucleus accumbens, bed nucleus of the stria terminalis, central nucleus of the amygdala, basolateral amygdala, hippocampal regions, and ventral tegmental area [2, 45, 68]. The dorsal striatum, that receives efferent projections from the mesocortical (the ventral tegmental area to prefrontal cortex) dopamine pathway, and the ventral striatum (nucleus accumbens) are considered the two focal points for reinstatement of drug-seeking behavior, or relapse centers of the brain [2, 68]. Most importantly, it is believed that the release of the neurotransmitters dopamine, glutamate, and corticotropin-releasing factor in these key brain regions are essential for the behavioral effects of the drug during the withdrawal and craving stages of addiction that contribute to relapse [72, 73]. The overlapping effects of exercise and methamphetamine on this neurocircuitry has been elegantly detailed in a recent review [7]; this overlap is briefly touched upon here from the perspectives of exercise addiction and of exercise therapy for methamphetamine addiction (Table 1).
To add to the existing theories on addiction, one recent discovery that is potentially important for addiction research is the ability of the adult mammalian brain to continuously generate new progenitors throughout adulthood. Broadly defined, progenitors are a progeny of stem cells that are characterized by limited self-renewal and can mature into differentiating cells, such as neurons and glia in the brain. The discovery [74–76] and eventual acceptance [77, 78] that adult-generated progenitors that can mature into neurons or glial cells in numerous mammalian species, including humans (for review [79]) has spurred substantial investigation of the proliferative capacity of brain regions such as thehippocampus. The role of adult neurogenesis in the hippocampus in methamphetamine addiction andthe influence of exercise on these neural progenitors will be discussed in the subsequent sections.
NEURAL PROGENITORS AND NEUROGENESIS IN THE HIPPOCAMPUS IS AFFECTED BY METHAMPHETAMINE AND EXERCISE
Adult neurogenesis in the adult mammalian brain occurs in only one region of the hippocampus, namely the subgranular zone, situated on the border of the granule cell layer and hilus of the dentate gyrus. Stem-like precursor cells are mitotically active and divide into preneuronal progenitors (while simultaneously maintaining the stem-like population) which then differentiate into mature granule cells [80–82]. There is strong evidence that these cells not only functionally integrate into the hippocampal network [82–89], but also regulate hippocampal-sensitive cognitive function [90–100]. Further, the neurogenic properties of the dentate gyrus can be modulated by multiple factors, including physical activity and drugs of abuse [101, 102].
REGULATION OF HIPPOCAMPAL NEUROGENESIS BY METHAMPHETAMINE
The ability of methamphetamine to modulate proliferation and survival of hippocampal neural progenitors has not been as prolific of a research focus. Of the few studies investigating the deleterious impact of methamphetamine on dentate gyrus neuronal generation, both acute administrations [103, 104] and chronic exposures [105] reduce proliferation and survival of neural progenitors, although intermittent access to the drug appears to be unique in its enhancement of proliferation of progenitors [105, 106]. Further, findings from a recent cell cycle kinetic study (where newly born proliferating cells were sequentially labeled with mitotic markers to calculate the rate of movement of progenitors through the cell cycle) determined that the impairment of proliferation following chronic methamphetamine self-administration was the result of a reduction in number of S-phase progenitors (as opposed to a change in the length of S-phase of the cell cycle) [106]. With regards to hippocampal cognitive performance following methamphetamine self-administration, evidence demonstrates that performance on spatial and working memory tasks are inversely correlated to the quantity of methamphetamine consumed (operant self-administration) which also negatively correlates with hippocampal proliferation [107]. Interestingly, the survival of progenitors birth-dated at the start of the abstinent period was increased and positively correlated with reinstatement of methamphetamine seeking, thus implying a relationship between the survival of neural progenitors and the severity of relapse to the drug [107]. One potential mechanism for the impairments in hippocampal granule cell proliferation is the observed dysregulation of the trophic factor brain derived neurotrophic factor (BDNF) and its receptor Tropomyosin receptor kinase B (TrkB), along with compromised glutamatergic signaling [108]. Taken together, these findings serve to support the theory that the neurogenic dysregulation caused by methamphetamine is an additional source of vulnerability to dependence on the drug [109–111].
REGULATION OF HIPPOCAMPAL NEUROGENESIS BY EXERCISE
Exercise exerts beneficial effects on hippocampal function and neurogenesis. Extensive evidence has demonstrated that exercise in animals is correlated with increases in both the number of neural progenitors as well as the number of surviving neurons in the hippocampus (for review, [56]). One of the mechanisms suggested for this increased neurogenesis is exercise-mediated increases in hippocampal trophic factors that are generally believed to support neuronal proliferation and survival, including BDNF and its receptor TrkB [112–128]. Other factors underlying the increased neurogenesis in the granule cell layer include increased stimulation and network activity in the surrounding hippocampus [115, 117, 119, 122, 124, 129–132], immune system regulation [133–136], and modulation of stress hormones [116, 137–140]. Furthermore, these increases in granule cell production correspond to improvements in hippocampal sensitive tasks including spatial navigation, pattern separation, and contextual fear conditioning (for review, [141]). Interestingly, evidence suggests that while the proliferative stimulation following exercise is lost relatively soon following cessation of activity, the increase in survival of granule cells continues for an extended duration, including into advanced age [58, 142]. Taken together, physical activity clearly enhances hippocampal neurogenesis and subsequently, hippocampal dependent cognition.
EXERCISE-INDUCED REDUCTION OF METHAMPHETAMINE TAKING AND SEEKING IS NOT ASSOCIATED WITH EXERCISE-INDUCED ENHANCEMENT OF HIPPOCAMPAL PROGENITORS AND NEUROGENESIS
It has been hypothesized that exercise-induced upregulation of hippocampal neurogenesis may underlie exercise-mediated reduction in the vulnerability to relapse in methamphetamine addicted animals [101]. However, recent findings demonstrate that wheel running-induced reduction in escalation of methamphetamine self-administration and reduction in reinstatement of methamphetamine seeking triggered by drug context and drug cues occurred independent of alterations in the number of neural progenitors and neurogenesis in the hippocampus [58, 59]. Therefore, while exercise and methamphetamine exert seemingly opposite effects on hippocampal neurogenesis (Fig. 1), the ability of exercise to regulate methamphetamine seeking appears to be dissociated from their effects of neurogenic events. Nevertheless, these studies have demonstrated that running activity during methamphetamine-self administration and during withdrawal prevented other measures of toxicity produced by methamphetamine (discussed below) to inhibit methamphetamine seeking behaviors [59].
METHAMPHETAMINE PRODUCES TOXICITY IN THE BRAIN
Studies over the past four decades have conceptualized that methamphetamine produces significant toxicity in the striatum, and that the neurotoxicity is responsible for the addictive effects of the drug. Briefly, methamphetamine reduces the function of dopamine transporters, vesicular monoamine transporters, tyrosine hydroxylase and MAO-B, to produce excessive dopamine release [143, 144] which leads to striatal neurotoxicity via enhanced reactive oxygen species (ROS) and reactive nitrogen species [145–157], and via compromised mitochondrial function [158, 159]. Methamphetamine also increases mitochondria-dependent pro-apoptotic proteins, decreases mitochondria-dependent anti-apoptotic proteins, and damages the endoplasmic reticulum, all of which contribute to increased neuronal cell death [159, 160]. Taken together, increased methamphetamine intake during extended access schedules of reinforcement leads to striatal neurotoxicity, which may be critical for the transition to compulsive use [161].
Non-striatal mechanisms have also been suggested for the emergence of the relapse/preoccupation anticipation stage of methamphetamine addiction [2]. Human imaging studies and postmortem studies provide converging evidence suggesting that chronic methamphetamine use produces neurotoxicity in the hippocampus [162, 163], and reduces frontal cortical, amygdalar, as well as hippocampal gray matter volumes [164–170], all of which may underlie the profound deficits in executive function, declarative memory, and impulsivity in methamphetamine addicts [170–172]. The behavioral deficits observed in methamphetamine addicts have been demonstrated in preclinical models of binge methamphetamine exposure and extended access methamphetamine self-administration, where methamphetamine exposure produces cognitive dysfunction and memory impairments dependent on the hippocampus [48, 107, 173–176]. Neurodegeneration and neurotoxicity in the hippocampus is also observed, and positively correlated with methamphetamine-induced behavioral deficits [105, 177–179]. Additional mechanistic studies show that repeated methamphetamine exposure (via experimenter delivered paradigms) reduced the long-term potentiation of hippocampal CA1 pyramidal neurons [180, 181], increases excitability of dentate gyrus neurons [182], demonstrating that methamphetamine exposure produces synaptic maladaptation in the hippocampus that may mediate some of the addiction-dependent behaviors. Since long-term potentiation in CA1 and dentate gyrus regions positively and bi-directionally interacts with hippocampal neurogenesis [122, 130, 132, 183–185], methamphetamine-mediated altered plasticity in these regions may contribute to methamphetamine’s effects on neurogenesis detailed in the section above.
Inflammation is another mechanism involved in methamphetamine-induced neurotoxicity, and its effects are considerably potentiated by oxidative stress [150]. Methamphetamine releases pro-inflammatory cytokines via activation of microglia and astroglia [186–188], which contribute to neuronal damage as well as behavioral deficits observed with chronic methamphetamine use [162, 189, 190]. Reduction in glial cell activation as well as administration of anti-inflammatory glial modulators have been found to reduce the sensitization to methamphetamine and methamphetamine self-administration in rodent models [191–194]. In conclusion, oxidative stress and associated mechanisms play a critical role in methamphetamine induced neurotoxicity and behavioral changes, which in turn further perpetuates maladaptive methamphetamine taking behavior.
PHYSICAL ACTIVITY REDUCES TOXICITY IN THE BRAIN
The previous sections have focused on the detrimental effects of ROS, however, it is important to understand that moderate levels of reactive species are in fact, beneficial and essential for cellular signaling, and epigenetic regulation [195]. Moderate levels of exercise regulates the body’s redox homeostasis by improving metabolism and blood flow throughout the body, and that contributes to the several health promoting effects of exercise (for review [195]). Specifically, moderate exercise increases both antioxidant capacity and resistance against oxidative stress throughout the body, thus increasing resistance and tolerance to oxidative stress. Interestingly, the brain appears to be particularly protected against the adverse oxidative effects of over (or exhaustive)-exercise [196–199]. Specifically, the brain remained protected while skeletal muscles, liver and kidney showed oxidative damage following a single bout of exhaustive exercise; where the exercise paradigm involved treadmill running at 24 meters/min until the rat lost its righting reflex [196, 197]. Also, chronic exercise did not increase lipid peroxidation in the rat brain [198], although these effects are modulated by dietary vitamin intake [200, 201]. Compared to moderate training (swimming1 hr/day for 8 weeks), very hard training (progressively increasing swim-time to 4.5 hr/day) and over-training (abruptly increasing swim-time from 1 hr/day to4.5 hr/day) decreased brain protein oxidation products and improved performance in an associated rat memory task [199]; however, this tolerance has its limits as others report increased ROS and lipid peroxidation and impaired memory following intense or exhaustive exercise [202–204]. Exercise has been shown to exert beneficial effects in rodent models of several brain disorders including, but not limited to, models of ischemic stroke, stroke-prone spontaneously hypertensive rats and N-methyl-D-aspartate (NMDA) lesion models for Alzheimer’s disease [205–207].
Several sub-cellular mechanisms are suggested to underlie the effects of exercise on brain function. These include increased expression of brain anti-oxidants including several superoxide dismutases, glutathione peroxidase and peroxisome proliferator-activated receptor-γ coactivator 1α (PGC-1α) in the striatum and the hippocampus [208, 209]. The latter transcription factor, PGC-1α, is doubly beneficial as it can further upregulate other antioxidant enzymes, and more importantly, increase biogenesis of mitochondria to improve the efficiency of ATP generation, and thereby, reduce oxidative neurodegeneration in the substantia nigra and the hippocampus [210]. Exercise is also reported to increase the activity of proteasome complex in the brain [198], which is a putative mechanism for improved cognition and reduced neurodegeneration in Alzheimer’s Disease and Parkinson’s Disease [211, 212].
Exercise, via modulation of oxidative pathways as well as through independent mechanisms, has been shown to modulate trophic factor signaling in the brain to promote brain plasticity (for review, [3, 213]). Specifically, exercise increases hippocampal BDNF expression, activates its downstream signaling pathways, and increases cAMP response element binding protein (CREB), that subsequently upregulates other inducible transcription factors [214–216]. These molecular changes could manifest themselves as beneficial neuroplasticity changes such as increasing cell survival [217, 218], formation of new synapses (synaptogenesis; [219]), and neurogenesis [220, 221]. Furthermore, because ROS is known to dose-dependently modulate proliferation of neuronal progenitor cells in the hippocampus [217, 222], exercise may modulate neurogenesis by regulating ROS in the dentate gyrus. Modulating the levels of trophic factors and ROS in regions other than the dentate gyrus may alter the generation of new glia (gliogenesis), such as astroglia and oligodendroglia that may contribute to functional plasticity [222–225]. The medial prefrontal cortex, a brain region involved in executive and impulse control [38, 226, 227], is one region where this mechanism has been investigated [44]. Specifically, voluntary wheel running in rats was also shown to increase in proliferation and survival of progenitor cells medial prefrontal cortex, and these new cells matured into new astroglia (∼33% ) and new oligodendroglia (∼55% ) [44]. Thus, exercise-induced gliogenesis may be a mechanism underlying exercise-induced improvement in cognition and executive function observed in several disease conditions [228, 229].
EXERCISE-INDUCED REDUCTION OF METHAMPHETAMINE TAKING AND SEEKING IS ASSOCIATED WITH EXERCISE-INDUCED REDUCTION OF METHAMPHETAMINE-INDUCED BRAIN TOXICITY
As discussed previously, exercise reduces methamphetamine intake when both the reinforcers are concurrently available, in part, because exercise serves as an alternative reinforcer ([7]; also see Table 1). However, the above sections demonstrate that the potential for overlap between exercise and methamphetamine extends much deeper and is more complicated than simply modulating neurotransmission in the brain reward and reinstatement centers (Fig. 1). Exercise improves brain function via changes in redox homeostasis and contributes to increased resistance and tolerance to oxidative challenge, and thereby promotes cell survival. Notably, swimming exercise was found to attenuate amphetamine-induced CPP and anxiety, which corresponded with exercise-induced reduction in ROS and protein oxidation products in hippocampal homogenates [54]. Studies conducted by our group and others revealed that rats with continued access to voluntary wheel running attenuated acquisition and escalation of methamphetamine self-administration compared to rats without access to running wheels [58, 66]. Furthermore, access to running wheels during protracted abstinence from methamphetamine accelerated extinction and attenuated reinstatement of methamphetamine seeking [59]. Indeed, voluntary running attenuated methamphetamine-induced damage to dopamine and serotonin terminals in the striatum, potentially, by promoting repair of the damaged terminals [230, 231]. Further, attenuated methamphetamine self-administration in rats with continued access to voluntary wheel running was associated with attenuation of neuronal apoptosis and decreased neuronal nitric oxide synthase expression in the nucleus accumbens [58], suggesting that regulation of oxidative stress mechanisms may be an alternate or concomitant mechanism which contributes to the beneficial effects of exercise in methamphetamine addiction. Future studies should include empirical validation of these mechanisms in exercise-mediated modulation of methamphetamine seeking.
Interestingly, withdrawal from wheel running during methamphetamine self-administration enhanced consumption of methamphetamine compared to exercise-naïve rats [58], suggesting that deprivation from chronic exercise may render subjects vulnerable to methamphetamine. The latter observation further contributes to the idea that exercise itself is addictive, however, very little is known about the mechanism underlying this increased addiction vulnerability. Evidence from studies in mice selectively bred for increased voluntary wheel running suggests dopamine is increased in the nucleus accumbens and the dorsal striatum (the reward and reinforcement centers of the brain), but is negatively correlated with running suggesting attenuation of sensitivity to reinforcing effects of running [232]. Furthermore, the dorsal striatum and substantia nigra were hypo-dopaminergic [233] thus providing a conducive environment for mechanisms that can compensate for this reduced dopamine tone (for example, methamphetamine-induced dopaminerelease; Table 1). Finally, running correlated with hippocampal activation (increased Fos) in controls but this correlation was lost in mice selectively bred for high wheel running activity [234]. Instead, the high runners showed increased activation (Fos) of the motivation circuitry, i.e. prefrontal cortex, striatum and lateral hypothalamus along with increased BDNF in the hippocampus [234, 235]. Taken together, a blunted dopamine reward system, a hypo-dopaminergic response in the nigro-striatal pathway (relapse circuitry) together with the activation of neurons in the brain regions involved in motivation in the exercise-withdrawn animal, may contribute to the addictive effects of exercise and may provide the ideal environment for cross-sensitization to other powerful reinforcers such as methamphetamine. However, these speculations need to be empirically validated using animal models.
FUTURE DIRECTIONS AND CONCLUSION
While some information is available regarding the associated neurobiological changes underlying the interactions of exercise with methamphetamine taking and seeking, a lot of the potential mechanisms have not been empirically evaluated. An example, is the opposite effects of methamphetamine and chronic exercise on astrocytes and inflammatory markers in the brain [186, 187, 236, 237], and yet their interaction in these avenues remains unexplored. Furthermore, there is a gap in our understanding of the neurobiological adaptations following exercise withdrawal and neurobehavioral effects of excessive exercise. An emerging direction in drug abuse research investigates epigenetic modifications of the chromatin structure that results in activation or repression of gene expression in altering the vulnerability to drug addiction [238, 239]. Epigenetic modification not only alters the rewarding effects of methamphetamine, chronic methamphetamine also produces epigenetic changes that are permissive for progressing through the different phases of addiction [240, 241]. Particularly, modifications in the genes regulating BDNF expression and signaling have been implicated in the initiation-binge/intoxication stage as well as the relapse-preoccupation/anticipation stage of psychostimulant addiction (for review, [242]). Exercise is known to increase BDNF expression in the hippocampus [243], and epigenetic modifications have been shown to be a part of the underlying mechanism [244]. It would be interesting to see how epigenetic modifications by exercise lead to long-term adaptations that promote/inhibit methamphetamine taking and seeking.
In sum, the identification of the neuroplastic and neuromodulatory effects of exercise over the past few decades has shed new light on the therapeutic and, more recently, the protective effects that are associated with exercise. Future studies aimed at understanding the potential link between correlative decreases in illicit drug use and addiction and increase in exercise output in animal models will allow researchers to determine whether decreases in neurotoxicity by exercise is behaviorally relevant to the prevention of addiction to illicit drugs. This may pave the way for future therapeutic possibilities for treating drug addiction disorders.
ACKNOWLEDGMENTS
Preparation of this review was supported by funds from the National Institute on Drug Abuse (DA034140 to CDM) and the National Institute on Alcohol Abuse and Alcoholism (AA020098 and AA006420 to CDM, and T32AA00747 to MCS). We appreciate the assistance of Janet Hightower from Biomedical Graphics Department at Scripps for assistance with the schematic presentation. This is publication number 29064 from the Scripps Research Institute.
REFERENCES
1 | NIDA. Topics in brief: Methamphetamine addiction: Progress, but need to remain vigilant. The Science of Drug Abuse and Addiction. 2011 |
2 | Koob GF, Volkow ND(2010) Neurocircuitry of addictionNeuropsychopharmacology35: 1217238 |
3 | Phillips C, Baktir MA, Srivatsan M, Salehi A(2014) Neuroprotective effects of physical activity on the brain: A closer look at trophic factor signalingFrontiers in Cellular Neuroscience8: 170 |
4 | Koob GF, Le Moal M(1997) Drug abuse: Hedonic homeostatic dysregulationScience278: 53355258 |
5 | Crews FT, Boettiger CA(2009) Impulsivity, frontal lobes and risk for addictionPharmacol Biochem Behav93: 3237247 |
6 | Feltenstein MW, See RE(2008) The neurocircuitry of addiction: An overviewBr J Pharmacol154: 2261274 |
7 | Lynch WJ, Peterson AB, Sanchez V, Abel J, Smith MA(2013) Exercise as a novel treatment for drug addiction: A neurobiological and stage-dependent hypothesisNeurosci Biobehav Rev37: 816221644 |
8 | Kanarek RB, D’Anci KE, Jurdak N, Mathes WF(2009) Running and addiction: Precipitated withdrawal in a rat model of activity-based anorexiaBehav Neurosci123: 4905912 |
9 | Carroll ME, Boe IN(1982) Increased intravenous drug self-administration during deprivation of other reinforcersPharmacol Biochem Behav17: 3563567 |
10 | SAMHSA. Results from the 2007 National Survey on Drug Use and Health: Detailed Tables. Substance Abuse and Mental Health Services Administration, Office of Applied Studies. 2008 |
11 | (USPSTF) USPSTF. Screening for illicit drug use. 2008 |
12 | (WHO) WHO. 1980 |
13 | NIDA. Research reports: Methamphetamine abuse and addiction. The Science of Drug Abuse and Addiction. 2006 |
14 | NIDA. Drug Facts: Methamphetamine. The Science of Drug Abuse and Addiction. 2010 |
15 | Gonzales R, Mooney L, Rawson RA(2010) The methamphetamine problem in the United StatesAnnu Rev Public Health31: 385398 |
16 | Rawson RA, Shoptaw SJ, Obert JL, McCann MJ, Hasson AL, Marinelli-Casey PJ(1995) An intensive outpatient approach for cocaine abuse treatment. The Matrix modelJ Subst Abuse Treat12: 2117127 |
17 | Gettig JP, Grady SE, Nowosadzka I(2006) Methamphetamine: Putting the brakes on speedJ Sch Nurs22: 26673 |
18 | Barrett B, Hayney MS, Muller D, Rakel D, Ward A, Obasi CN(2012) Meditation or exercise for preventing acute respiratory infection: A randomized controlled trialAnnals of family medicine10: 4337346 |
19 | Wang G, Pratt M, Macera CA, Zheng ZJ, Heath G(2004) Physical activity, cardiovascular disease, and medical expenditures in U.S. adultsAnnals of behavioral medicine: A publication of the Society of Behavioral Medicine28: 28894 |
20 | Pratt M, Macera CA, Wang G(2000) Higher direct medical costs associated with physical inactivityThe Physician and sportsmedicine28: 106370 |
21 | Patel AV, Bernstein L, Deka A, Feigelson HS, Campbell PT, Gapstur SM(2010) Leisure time spent sitting in relation to total mortality in a prospective cohort of US adultsAm J Epidemiol172: 4419429 |
22 | United States. Department of Health and Human Services. 2008 physical activity guidelines for Americans: Be active, healthy, and happy! Washington, DC: U.S. Dept. of Health and Human Services; 2008. ix, pp. 61 |
23 | Mekary RA, Feskanich D, Hu FB, Willett WC, Field AE(2010) Physical activity in relation to long-term weight maintenance after intentional weight loss in premenopausal womenObesity (Silver Spring)18: 1167174 |
24 | DiLorenzo TM, Bargman EP, Stucky-Ropp R, Brassington GS, Frensch PA, LaFontaine T(1999) Long-term effects of aerobic exercise on psychological outcomesPrev Med28: 17585 |
25 | Blumenthal JA, Babyak MA, Moore KA, Craighead WE, Herman S, Khatri P(1999) Effects of exercise training on older patients with major depressionArch Intern Med159: 1923492356 |
26 | Peluso MA, Guerra de Andrade LH(2005) Physical activity and mental health: The association between exercise and moodClinics60: 16170 |
27 | Koob GF(2013) Addiction is a Reward Deficit and Stress Surfeit DisorderFrontiers in Psychiatry4: 72 |
28 | Jones EM, Knutson D, Haines D(2003) Common problems in patients recovering from chemical dependencyAmerican Family Physician68: 1019711978 |
29 | Polter AM, Kauer JA(2014) Stress and VTA synapses: Implications for addiction and depressionEur J Neurosci39: 711791188 |
30 | Egorov AY, Szabo A(2013) The exercise paradox: An interactional model for a clearer conceptualization of exercise addictionJournal of Behavioral Addictions2: 4199208 |
31 | American Psychiatric Association., American Psychiatric Association. DSM-5 Task Force. Diagnostic and statistical manual of mental disorders: DSM-5. 5th ed. Washington, D.C.: American Psychiatric Publishing; 2013. xliv, pp. 947 |
32 | Sabzo A(2010) Addiction to Exercise: A Symptom or a Disorder?New YorkNova Science Publishers Inc |
33 | Bruno A, Quattrone D, Scimeca G, Cicciarelli C, Romeo VM, Pandolfo G(2014) Unraveling exercise addiction: The role of narcissism and self-esteemJournal of Addiction2014: 987841 |
34 | Meulemans S, Pribis P, Grajales T, Krivak G(2014) Gender differences in exercise dependence and eating disorders in young adults: A path analysis of a conceptual modelNutrients6: 1148954905 |
35 | Council on Clinical Classifications., National Center for Health Statistics (U.S.), World Health Organization., Commission on Professional and Hospital Activities. The International classification of diseases, clinical modifications, ICD-9-CM. 9th revision. ed. Ann Arbor: CPHA; 1978 |
36 | Pope HGJr, Gruber AJ, Choi P, Olivardia R, Phillips KA(1997) Muscle dysmorphia. An underrecognized form of body dysmorphic disorderPsychosomatics38: 6548557 |
37 | Lichtenstein MB, Andries A, Hansen S, Frystyk J, Stoving RK(2015) Exercise addiction in men is associated with lower fat-adjusted leptin levelsClinical Journal of Sport Medicine: Official Journal of the Canadian Academy of Sport Medicine25: 2138143 |
38 | Kalivas PW, Volkow ND(2005) The neural basis of addiction: A pathology of motivation and choiceAm J Psychiatry162: 814031413 |
39 | Haglund M, Ang A, Mooney L, Gonzales R, Chudzynski J, Cooper CB(2014) Predictors of depression outcomes among abstinent methamphetamine-dependent individuals exposed to an exercise interventionAm J Addict |
40 | Muller AE, Clausen T(2014) Group exercise to improve quality of life among substance use disorder patientsScandinavian Journal of Public Health |
41 | Ahmed SH, Koob GF(1998) Transition from moderate to excessive drug intake: Change in hedonic set pointScience282: 5387298300 |
42 | Gawin FH, Ellinwood EHJr(1989) Cocaine dependenceAnnu Rev Med40: 149161 |
43 | Kramer JC, Fischman VS, Littlefield DC(1967) Amphetamine abuse. Pattern and effects of high doses taken intravenouslyJama201: 5305309 |
44 | Mandyam CD, Wee S, Eisch AJ, Richardson HN, Koob GF(2007) Methamphetamine self-administration and voluntary exercise have opposing effects on medial prefrontal cortex gliogenesisJ Neurosci27: 421144211450 |
45 | Shaham Y, Shalev U, Lu L, De Wit H, Stewart J(2003) The reinstatement model of drug relapse: History, methodology and major findingsPsychopharmacology (Berl)168: 1-2320 |
46 | Shalev U, Grimm JW, Shaham Y(2002) Neurobiology of relapse to heroin and cocaine seeking: A reviewPharmacol Rev54: 1142 |
47 | McFarland K, Kalivas PW(2001) The circuitry mediating cocaine-induced reinstatement of drug-seeking behaviorJ Neurosci21: 2186558663 |
48 | Rogers JL, De Santis S, See RE(2008) Extended methamphetamine self-administration enhances reinstatement of drug seeking and impairs novel object recognition in ratsPsychopharmacology (Berl)199: 4615624 |
49 | Spragg SDS(1940) Morphine addiction in chimpanzeesComparative Psychology Monographs15: 1132 |
50 | Bardo MT, Bevins RA(2000) Conditioned place preference: What does it add to our preclinical understanding of drug reward? Psychopharmacology (Berl)153: 13143 |
51 | Advokat C(1985) Evidence of place conditioning after chronic intrathecal morphine in ratsPharmacol Biochem Behav22: 2271277 |
52 | Bardo MT, Miller JS, Neisewander JL(1984) Conditioned place preference with morphine: The effect of extinction training on the reinforcing CRPharmacol Biochem Behav21: 4545549 |
53 | Arida RM, Scorza FA, Gomes da Silva S, Cysneiros RM, Cavalheiro EA(2011) Exercise paradigms to study brain injury recovery in rodentsAmerican Journal of Physical Medicine & Rehabilitation / Association of Academic Physiatrists90: 6452465 |
54 | Segat HJ, Kronbauer M, Roversi K, Schuster AJ, Vey LT, Roversi K(2014) Exercise modifies amphetamine relapse: Behavioral and oxidative markers in ratsBehav Brain Res262: 94100 |
55 | Rupp H, Wahl R(1990) Influence of thyroid hormones and catecholamines on myosin of swim-exercised ratsJournal of Applied Physiology68: 3973978 |
56 | Voss MW, Vivar C, Kramer AF, van Praag H(2013) Bridging animal and human models of exercise-induced brain plasticityTrends in Cognitive Sciences17: 10525544 |
57 | Greenwood BN, Foley TE, Le TV, Strong PV, Loughridge AB, Day HE(2011) Long-term voluntary wheel running is rewarding and produces plasticity in the mesolimbic reward pathwayBehavioural Brain Research217: 2354362 |
58 | Engelmann AJ, Aparicio MB, Kim A, Sobieraj JC, Yuan CJ, Grant Y(2014) Chronic wheel running reduces maladaptive patterns of methamphetamine intake: Regulation by attenuation of methamphetamine-induced neuronal nitric oxide synthaseBrain Struct Funct219: 2657672 |
59 | Sobieraj JC, Kim A, Fannon MJ, Mandyam CD(2014) Chronic wheel running-induced reduction of extinction and reinstatement of methamphetamine seeking in methamphetamine dependent rats is associated with reduced number of periaqueductal gray dopamine neuronsBrain Struct Funct |
60 | Ferreira A, Lamarque S, Boyer P, Perez-Diaz F, Jouvent R, Cohen-Salmon C(2006) Spontaneous appetence for wheel-running: A model of dependency on physical activity in ratEur Psychiatry21: 8580588 |
61 | Brene S, Bjornebekk A, Aberg E, Mathe AA, Olson L, Werme M(2007) Running is rewarding and antidepressivePhysiol Behav92: 1-2136140 |
62 | Kolb EM, Kelly SA, Garland TJr(2013) Mice from lines selectively bred for high voluntary wheel running exhibit lower blood pressure during withdrawal from wheel accessPhysiology & Behavior112-113: 4955 |
63 | Perrine SA, Sheikh IS, Nwaneshiudu CA, Schroeder JA, Unterwald EM(2008) Withdrawal from chronic administration of cocaine decreases delta opioid receptor signaling and increases anxiety- and depression-like behaviors in the ratNeuropharmacology54: 2355364 |
64 | Anraku T, Ikegaya Y, Matsuki N, Nishiyama N(2001) Withdrawal from chronic morphine administration causes prolonged enhancement of immobility in rat forced swimming testPsychopharmacology157: 2217220 |
65 | Malisch JL, Breuner CW, Kolb EM, Wada H, Hannon RM, Chappell MA(2009) Behavioral despair and home-cage activity in mice with chronically elevated baseline corticosterone concentrationsBehavior Genetics39: 2192201 |
66 | Miller ML, Vaillancourt BD, Wright MJJr, Aarde SM, Vandewater SA, Creehan KM(2012) Reciprocal inhibitory effects of intravenous d-methamphetamine self-administration and wheel activity in ratsDrug Alcohol Depend121: 1-29096 |
67 | Fontes-Ribeiro CA, Marques E, Pereira FC, Silva AP, Macedo TR(2011) May exercise prevent addiction?Curr Neuropharmacol9: 14548 |
68 | Crombag HS, Bossert JM, Koya E, Shaham Y(2008) Review. Context-induced relapse to drug seeking: A reviewPhilos Trans R Soc Lond B Biol Sci363: 150732333243 |
69 | Floresco SB, Todd CL, Grace AA(2001) Glutamatergic afferents from the hippocampus to the nucleus accumbens regulate activity of ventral tegmental area dopamine neuronsJ Neurosci21: 1349154922 |
70 | Floresco SB, Blaha CD, Yang CR, Phillips AG(2001) Modulation of hippocampal and amygdalar-evoked activity of nucleus accumbens neurons by dopamine: Cellular mechanisms of input selectionJ Neurosci21: 828512860 |
71 | Taepavarapruk P, Floresco SB, Phillips AG(2000) Hyperlocomotion and increased dopamine efflux in the rat nucleus accumbens evoked by electrical stimulation of the ventral subiculum: Role of ionotropic glutamate and dopamine D1 receptorsPsychopharmacology (Berl)151: 2-3242251 |
72 | Koob GF(1999) Stress, corticotropin-releasing factor, and drug addictionAnn N Y Acad Sci897: 2745 |
73 | Knackstedt LA, Kalivas PW(2009) Glutamate and reinstatementCurr Opin Pharmacol9: 15964 |
74 | Messier B, Leblond CP(1960) Cell proliferation and migration as revealed by radioautography after injection of thymidine-H3 into male rats and miceAm J Anat106: 247285 |
75 | Altman J(1969) Autoradiographic and histological studies of postnatal neurogenesis. 3. Dating the time of production and onset of differentiation of cerebellar microneurons in ratsJ Comp Neurol136: 3269293 |
76 | Altman J, Das GD(1965) Autoradiographic and histological evidence of postnatal hippocampal neurogenesis in ratsJ Comp Neurol124: 3319335 |
77 | Eriksson PS, Perfilieva E, Bjork-Eriksson T, Alborn AM, Nordborg C, Peterson DA(1998) Neurogenesis in the adult human hippocampusNat Med4: 1113131317 |
78 | Manganas LN, Zhang X, Li Y, Hazel RD, Smith SD, Wagshul ME(2007) Magnetic resonance spectroscopy identifies neural progenitor cells in the live human brainScience318: 5852980985 |
79 | Bonfanti L, Peretto P(2011) Adult neurogenesis in mammals–a theme with many variationsEur J Neurosci34: 6930950 |
80 | Kempermann G, Jessberger S, Steiner B, Kronenberg G(2004) Milestones of neuronal development in the adult hippocampusTrends Neurosci27: 8447452 |
81 | Abrous DN, Koehl M, Le Moal M(2005) Adult neurogenesis: From precursors to network and physiologyPhysiol Rev85: 2523569 |
82 | Toni N, Laplagne DA, Zhao C, Lombardi G, Ribak CE, Gage FH(2008) Neurons born in the adult dentate gyrus form functional synapses with target cellsNat Neurosci11: 8901907 |
83 | Gould E, Reeves AJ, Fallah M, Tanapat P, Gross CG, Fuchs E(1999) Hippocampal neurogenesis in adult Old World primatesProceedings of the National Academy of Sciences of the United States of America96: 952635267 |
84 | Gould E, Reeves AJ, Graziano MS, Gross CG(1999) Neurogenesis in the neocortex of adult primatesScience286: 5439548552 |
85 | Shors TJ, Townsend DA, Zhao M, Kozorovitskiy Y, Gould E(2002) Neurogenesis may relate to some but not all types of hippocampal-dependent learningHippocampus12: 5578584 |
86 | Aimone JB, Wiles J, Gage FH(2006) Potential role for adult neurogenesis in the encoding of time in new memoriesNat Neurosci9: 6723727 |
87 | Clark PJ, Bhattacharya TK, Miller DS, Kohman RA, DeYoung EK, Rhodes JS(2012) New neurons generated from running are broadly recruited into neuronal activation associated with three different hippocampus-involved tasksHippocampus22: 918601867 |
88 | Lacefield CO, Itskov V, Reardon T, Hen R, Gordon JA(2012) Effects of adult-generated granule cells on coordinated network activity in the dentate gyrusHippocampus22: 1106116 |
89 | Gould E, Beylin A, Tanapat P, Reeves A, Shors TJ(1999) Learning enhances adult neurogenesis in the hippocampal formationNat Neurosci2: 3260265 |
90 | Shors TJ, Miesegaes G, Beylin A, Zhao M, Rydel T, Gould E(2001) Neurogenesis in the adult is involved in the formation of trace memoriesNature410: 6826372376 |
91 | Feng R, Rampon C, Tang YP, Shrom D, Jin J, Kyin M(2001) Deficient neurogenesis in forebrain-specific presenilin-1 knockout mice is associated with reduced clearance of hippocampal memory tracesNeuron32: 5911926 |
92 | Deisseroth K, Singla S, Toda H, Monje M, Palmer TD, Malenka RC(2004) Excitation-neurogenesis coupling in adult neural stem/progenitor cellsNeuron42: 4535552 |
93 | Schmidt-Hieber C, Jonas P, Bischofberger J(2004) Enhanced synaptic plasticity in newly generated granule cells of the adult hippocampusNature429: 6988184187 |
94 | Kim WR, Christian K, Ming GL, Song H(2012) Time-dependent involvement of adult-born dentate granule cells in behaviorBehavioural Brain Research227: 2470479 |
95 | Bakker A, Kirwan CB, Miller M, Stark CE(2008) Pattern separation in the human hippocampal CA3 and dentate gyrusScience319: 587016401642 |
96 | Clelland CD, Choi M, Romberg C, Clemenson GDJr, Fragniere A, Tyers P(2009) A functional role for adult hippocampal neurogenesis in spatial pattern separationScience325: 5937210213 |
97 | Sahay A, Scobie KN, Hill AS, O’Carroll CM, Kheirbek MA, Burghardt NS(2011) Increasing adult hippocampal neurogenesis is sufficient to improve pattern separationNature472: 7344466470 |
98 | Sahay A, Wilson DA, Hen R(2011) Pattern separation: A common function for new neurons in hippocampus and olfactory bulbNeuron70: 4582588 |
99 | McHugh TJ, Jones MW, Quinn JJ, Balthasar N, Coppari R, Elmquist JK(2007) Dentate gyrus NMDA receptors mediate rapid pattern separation in the hippocampal networkScience317: 58349499 |
100 | Aimone JB, Deng W, Gage FH(2011) Resolving new memories: A critical look at the dentate gyrus, adult neurogenesis, and pattern separationNeuron70: 4589596 |
101 | Mandyam CD, Koob GF(2012) The addicted brain craves new neurons: Putative role for adult-born progenitors in promoting recoveryTrends Neurosci35: 4250260 |
102 | van Praag H(2008) Neurogenesis and exercise: Past and future directionsNeuromolecular Med10: 2128140 |
103 | Teuchert-Noodt G, Dawirs RR, Hildebrandt K(2000) Adult treatment with methamphetamine transiently decreases dentate granule cell proliferation in the gerbil hippocampusJ Neural Transm107: 2133143 |
104 | Kochman LJ, Fornal CA, Jacobs BL(2009) Suppression of hippocampal cell proliferation by short-term stimulant drug administration in adult ratsEur J Neurosci29: 1121572165 |
105 | Mandyam CD, Wee S, Crawford EF, Eisch AJ, Richardson HN, Koob GF(2008) Varied access to intravenous methamphetamine self-administration differentially alters adult hippocampal neurogenesisBiol Psychiatry64: 11958965 |
106 | Yuan CJ, Quiocho JM, Kim A, Wee S, Mandyam CD(2011) Extended access methamphetamine decreases immature neurons in the hippocampus which results from loss and altered development of neural progenitors without altered dynamics of the S-phase of the cell cyclePharmacol Biochem Behav100: 198108 |
107 | Recinto P, Samant AR, Chavez G, Kim A, Yuan CJ, Soleiman M(2012) Levels of neural progenitors in the hippocampus predict memory impairment and relapse to drug seeking as a function of excessive methamphetamine self-administrationNeuropsychopharmacology37: 512751287 |
108 | Galinato MH, Orio L, Mandyam CD(2014) Methamphetamine differentially affects BDNF and cell death factors in anatomically defined regions of the hippocampusNeuroscience286c: 97108 |
109 | Canales JJ(2007) Adult neurogenesis and the memories of drug addictionEur Arch Psychiatry Clin Neurosci257: 5261270 |
110 | Canales JJ(2010) Comparative neuroscience of stimulant-induced memory dysfunction: Role for neurogenesis in the adult hippocampusBehav Pharmacol21: 5-6379393 |
111 | Chambers RA(2013) Adult hippocampal neurogenesis in the pathogenesis of addiction and dual diagnosis disordersDrug and alcohol dependence130: 1-3112 |
112 | Neeper SA, Gomez-Pinilla F, Choi J, Cotman C(1995) Exercise and brain neurotrophinsNature373: 6510109 |
113 | Neeper SA, Gomez-Pinilla F, Choi J, Cotman CW(1996) Physical activity increases mRNA for brain-derived neurotrophic factor and nerve growth factor in rat brainBrain Research726: 1-24956 |
114 | Berchtold NC, Chinn G, Chou M, Kesslak JP, Cotman CW(2005) Exercise primes a molecular memory for brain-derived neurotrophic factor protein induction in the rat hippocampusNeuroscience133: 3853861 |
115 | Molteni R, Ying Z, Gomez-Pinilla F(2002) Differential effects of acute and chronic exercise on plasticity-related genes in the rat hippocampus revealed by microarrayThe European Journal of Neuroscience16: 611071116 |
116 | Uysal N, Kiray M, Sisman A, Camsari U, Gencoglu C, Baykara B(2015) Effects of voluntary and involuntary exercise on cognitive functions, and VEGF and BDNF levels in adolescent ratsBiotechnic & Histochemistry: Official publication of the Biological Stain Commission90: 15568 |
117 | Ding Q, Ying Z, Gomez-Pinilla F(2011) Exercise influences hippocampal plasticity by modulating brain-derived neurotrophic factor processingNeuroscience192: 773780 |
118 | Sartori CR, Vieira AS, Ferrari EM, Langone F, Tongiorgi E, Parada CA(2011) The antidepressive effect of the physical exercise correlates with increased levels of mature BDNF, and proBDNF proteolytic cleavage-related genes, p11 and tPANeuroscience180: 918 |
119 | Vaynman S, Ying Z, Gomez-Pinilla F(2003) Interplay between brain-derived neurotrophic factor and signal transduction modulators in the regulation of the effects of exercise on synaptic-plasticityNeuroscience122: 3647657 |
120 | Hopkins ME, Nitecki R, Bucci DJ(2011) Physical exercise during adolescence versus adulthood: Differential effects on object recognition memory and brain-derived neurotrophic factor levelsNeuroscience194: 8494 |
121 | Marlatt MW, Potter MC, Lucassen PJ, van Praag H(2012) Running throughout middle-age improves memory function, hippocampal neurogenesis, and BDNF levels in female C57BL/6J miceDevelopmental Neurobiology72: 6943952 |
122 | Farmer J, Zhao X, van Praag H, Wodtke K, Gage FH, Christie BR(2004) Effects of voluntary exercise on synaptic plasticity and gene expression in the dentate gyrus of adult male Sprague-Dawley rats in vivo Neuroscience124: 17179 |
123 | Berchtold NC, Castello N, Cotman CW(2010) Exercise and time-dependent benefits to learning and memoryNeuroscience167: 3588597 |
124 | Liu YF, Chen HI, Wu CL, Kuo YM, Yu L, Huang AM(2009) Differential effects of treadmill running and wheel running on spatial or aversive learning and memory: Roles of amygdalar brain-derived neurotrophic factor and synaptotagmin IJ Physiol587: Pt 1332213231 |
125 | Vaynman S, Ying Z, Gomez-Pinilla F(2004) Exercise induces BDNF and synapsin I to specific hippocampal subfieldsJournal of Neuroscience Research76: 3356362 |
126 | Griffin EW, Bechara RG, Birch AM, Kelly AM(2009) Exercise enhances hippocampal-dependent learning in the rat: Evidence for a BDNF-related mechanismHippocampus19: 10973980 |
127 | O’Callaghan RM, Griffin EW, Kelly AM(2009) Long-term treadmill exposure protects against age-related neurodegenerative change in the rat hippocampusHippocampus19: 1010191029 |
128 | Wu CW, Chang YT, Yu L, Chen HI, Jen CJ, Wu SY(2008) Exercise enhances the proliferation of neural stem cells and neurite growth and survival of neuronal progenitor cells in dentate gyrus of middle-aged miceJournal of Applied Physiology (Bethesda, Md: 1985)105: 515851594 |
129 | O’Callaghan RM, Ohle R, Kelly AM(2007) The effects of forced exercise on hippocampal plasticity in the rat: A comparison of LTP, spatial- and non-spatial learningBehavioural Brain Research176: 2362366 |
130 | van Praag H, Christie BR, Sejnowski TJ, Gage FH(1999) Running enhances neurogenesis, learning, and long-term potentiation in miceProc Natl Acad Sci U S A96: 231342713431 |
131 | Vasuta C, Caunt C, James R, Samadi S, Schibuk E, Kannangara T(2007) Effects of exercise on NMDA receptor subunit contributions to bidirectional synaptic plasticity in the mouse dentate gyrusHippocampus17: 1212011208 |
132 | Snyder JS, Kee N, Wojtowicz JM(2001) Effects of adult neurogenesis on synaptic plasticity in the rat dentate gyrusJ Neurophysiol85: 624232431 |
133 | Speisman RB, Kumar A, Rani A, Foster TC, Ormerod BK(2013) Daily exercise improves memory, stimulates hippocampal neurogenesis and modulates immune and neuroimmune cytokines in aging ratsBrain, Behavior, and Immunity28: 2543 |
134 | Moon HY, Kim SH, Yang YR, Song P, Yu HS, Park HG(2012) Macrophage migration inhibitory factor mediates the antidepressant actions of voluntary exerciseProceedings of the National Academy of Sciences of the United States of America109: 321309413099 |
135 | Walton NM, Sutter BM, Laywell ED, Levkoff LH, Kearns SM, Marshall GP2nd(2006) Microglia instruct subventricular zone neurogenesisGlia54: 8815825 |
136 | Ziv Y, Ron N, Butovsky O, Landa G, Sudai E, Greenberg N(2006) Immune cells contribute to the maintenance of neurogenesis and spatial learning abilities in adulthoodNat Neurosci9: 2268275 |
137 | Yau SY, Li A, Zhang ED, Christie BR, Xu A, Lee TM(2014) Sustained running in rats administered corticosterone prevents the development of depressive behaviors and enhances hippocampal neurogenesis and synaptic plasticity without increasing neurotrophic factor levelsCell Transplantation23: 4-5481492 |
138 | Gregoire CA, Bonenfant D, Le Nguyen A, Aumont A, Fernandes KJ(2014) Untangling the influences of voluntary running, environmental complexity, social housing and stress on adult hippocampal neurogenesisPloS One9: 1e86237 |
139 | Kwon DH, Kim BS, Chang H, Kim YI, Jo SA, Leem YH(2013) Exercise ameliorates cognition impairment due to restraint stress-induced oxidative insult and reduced BDNF levelBiochemical and Biophysical Research Communications434: 2245251 |
140 | Li H, Liang A, Guan F, Fan R, Chi L, Yang B(2013) Regular treadmill running improves spatial learning and memory performance in young mice through increased hippocampal neurogenesis and decreased stressBrain Research1531: 18 |
141 | Yau SY, Gil-Mohapel J(2014) Physical exercise-induced adult neurogenesis: A good strategy to prevent cognitive decline in neurodegenerative diseases?2014: 403120 |
142 | Merkley CM, Jian C, Mosa A, Tan YF, Wojtowicz JM(2014) Homeostatic regulation of adult hippocampal neurogenesis in aging rats: Long-term effects of early exerciseFrontiers in Neuroscience8: 174 |
143 | Ares-Santos S, Granado N, Moratalla R(2013) The role of dopamine receptors in the neurotoxicity of methamphetamineJ Intern Med273: 5437453 |
144 | Fleckenstein AE, Volz TJ, Riddle EL, Gibb JW, Hanson GR(2007) New insights into the mechanism of action of amphetaminesAnnu Rev Pharmacol Toxicol47: 681698 |
145 | Itzhak Y(1997) Modulation of cocaine- and methamphetamine-induced behavioral sensitization by inhibition of brain nitric oxide synthaseJ Pharmacol Exp Ther282: 2521527 |
146 | Itzhak Y, Anderson KL, Ali SF(2004) Differential response of nNOS knockout mice to MDMA ("ecstasy")- and methamphetamine-induced psychomotor sensitization and neurotoxicityAnn N Y Acad Sci1025: 119128 |
147 | Itzhak Y, Gandia C, Huang PL, Ali SF(1998) Resistance of neuronal nitric oxide synthase-deficient mice to methamphetamine-induced dopaminergic neurotoxicityJ Pharmacol Exp Ther284: 310401047 |
148 | Itzhak Y, Martin JL, Ali SF(1999) Methamphetamine- and 1-methyl-4-phenyl- 1,2,3, 6-tetrahydropyridine-induced dopaminergic neurotoxicity in inducible nitric oxide synthase-deficient miceSynapse34: 4305312 |
149 | Itzhak Y, Martin JL, Ali SF(2000) Comparison between the role of the neuronal and inducible nitric oxide synthase in methamphetamine-induced neurotoxicity and sensitizationAnn N Y Acad Sci914: 104111 |
150 | Yamamoto BK, Bankson MG(2005) Amphetamine neurotoxicity: Cause and consequence of oxidative stressCrit Rev Neurobiol17: 287117 |
151 | Wang J, Xu W, Ali SF, Angulo JA(1139) Connection between the striatal neurokinin-1 receptor and nitric oxide formation during methamphetamine exposureAnn N Y Acad Sci164171 |
152 | Eyerman DJ, Yamamoto BK(2007) A rapid oxidation and persistent decrease in the vesicular monoamine transporter 2 after methamphetamineJ Neurochem103: 312191227 |
153 | Wang J, Angulo JA(2011) Synergism between methamphetamine and the neuropeptide substance P on the production of nitric oxide in the striatum of miceBrain Res1369: 131139 |
154 | Zhu J, Xu W, Wang J, Ali SF, Angulo JA(2009) The neurokinin-1 receptor modulates the methamphetamine-induced striatal apoptosis and nitric oxide formation in miceJ Neurochem111: 3656668 |
155 | Fleckenstein AE, Hanson GR(2003) Impact of psychostimulants on vesicular monoamine transporter functionEur J Pharmacol479: 1-3283289 |
156 | Fleckenstein AE, Gibb JW, Hanson GR(2000) Differential effects of stimulants on monoaminergic transporters: Pharmacological consequences and implications for neurotoxicityEur J Pharmacol406: 1113 |
157 | Shiba T, Yamato M, Kudo W, Watanabe T, Utsumi H, Yamada K(2011) In vivo imaging of mitochondrial function in methamphetamine-treated ratsNeuroimage57: 3866872 |
158 | Burrows KB, Gudelsky G, Yamamoto BK(2000) Rapidand transient inhibition of mitochondrial functionfollowing methamphetamine or 3,4-methylenedioxy-methamphetamine administrationEur J Pharmacol398: 11118 |
159 | Deng X, Cai NS, McCoy MT, Chen W, Trush MA, Cadet JL(2002) Methamphetamine induces apoptosis in an immortalized rat striatal cell line by activating the mitochondrial cell death pathwayNeuropharmacology42: 6837845 |
160 | Jayanthi S, Deng X, Noailles PA, Ladenheim B, Cadet JL(2004) Methamphetamine induces neuronal apoptosis via cross-talks between endoplasmic reticulum and mitochondria-dependent death cascadesFASEB J18: 2238251 |
161 | Everitt BJ, Belin D, Economidou D, Pelloux Y, Dalley JW, Robbins TW(2008) Review. Neural mechanisms underlying the vulnerability to develop compulsive drug-seeking habits and addictionPhilos Trans R Soc Lond B Biol Sci363: 150731253135 |
162 | Kitamura O, Takeichi T, Wang EL, Tokunaga I, Ishigami A, Kubo S(2010) Microglial and astrocytic changes in the striatum of methamphetamine abusersLeg Med (Tokyo)12: 25762 |
163 | Kitamura O(2009) Detection of methamphetamine neurotoxicity in forensic autopsy casesLeg Med (Tokyo)11 Suppl1: S63S65 |
164 | Morales AM, Lee B, Hellemann G, O’Neill J, London ED(2012) Gray-matter volume in methamphetamine dependence: Cigarette smoking and changes with abstinence from methamphetamineDrug Alcohol Depend125: 3230238 |
165 | Orikabe L, Yamasue H, Inoue H, Takayanagi Y, Mozue Y, Sudo Y(2011) Reduced amygdala and hippocampal volumes in patients with methamphetamine psychosisSchizophr Res132: 2-3183189 |
166 | Nakama H, Chang L, Fein G, Shimotsu R, Jiang CS, Ernst T(2011) Methamphetamine users show greater than normal age-related cortical gray matter lossAddiction106: 814741483 |
167 | Daumann J, Koester P, Becker B, Wagner D, Imperati D, Gouzoulis-Mayfrank E(2011) Medial prefrontal gray matter volume reductions in users of amphetamine-type stimulants revealed by combined tract-based spatial statistics and voxel-based morphometryNeuroimage54: 2794801 |
168 | Schwartz DL, Mitchell AD, Lahna DL, Luber HS, Huckans MS, Mitchell SH(2010) Global and local morphometric differences in recently abstinent methamphetamine-dependent individualsNeuroimage50: 413921401 |
169 | Kim YT, Lee JJ, Song HJ, Kim JH, Kwon DH, Kim MN(2010) Alterations in cortical activity of male methamphetamine abusers performing an empathy task: fMRI studyHum Psychopharmacol25: 16370 |
170 | Thompson PM, Hayashi KM, Simon SL, Geaga JA, Hong MS, Sui Y(2004) Structural abnormalities in the brains of human subjects who use methamphetamineJ Neurosci24: 2660286036 |
171 | Price KL, DeSantis SM, Simpson AN, Tolliver BK, McRae-Clark AL, Saladin ME(2011) The impact of clinical and demographic variables on cognitive performance in methamphetamine-dependent individuals in rural South CarolinaAm J Addict20: 5447455 |
172 | Weber E, Blackstone K, Iudicello JE, Morgan EE, Grant I, Moore DJ(2012) Neurocognitive deficits are associated with unemployment in chronic methamphetamine usersDrug Alcohol Depend125: 1-2146153 |
173 | Itoh K, Fukumori R, Suzuki Y(1984) Effect of methamphetamine on the locomotor activity in the 6-OHDA dorsal hippocampus lesioned ratLife Sci34: 9827833 |
174 | Yoshikawa T, Shibuya H, Kaneno S, Toru M(1991) Blockade of behavioral sensitization to methamphetamine by lesion of hippocampo-accumbal pathwayLife Sci48: 1313251332 |
175 | Yamamoto J(1997) Cortical and hippocampal EEG power spectra in animal models of schizophrenia produced with methamphetamine, cocaine, and phencyclidinePsychopharmacology (Berl)131: 4379387 |
176 | Friedman SD, Castaneda E, Hodge GK(1998) Long-term monoamine depletion, differential recovery, and subtle behavioral impairment following methamphetamine-induced neurotoxicityPharmacol Biochem Behav61: 13544 |
177 | Commins DL, Seiden LS(1986) Alpha-Methyltyrosine blocks methylamphetamine-induced degeneration in the rat somatosensory cortexBrain Res365: 11520 |
178 | Eisch AJ, O’Dell SJ, Marshall JF(1996) Striatal and cortical NMDA receptors are altered by a neurotoxic regimen of methamphetamineSynapse22: 3217225 |
179 | Schmued LC, Bowyer JF(1997) Methamphetamine exposure can produce neuronal degeneration in mouse hippocampal remnantsBrain Res759: 1135140 |
180 | Swant J, Chirwa S, Stanwood G, Khoshbouei H(2010) Methamphetamine reduces LTP and increases baseline synaptic transmission in the CA1 region of mouse hippocampusPLoS ONE5: 6e11382 |
181 | Onaivi ES, Ali SF, Chirwa SS, Zwiller J, Thiriet N, Akinshola BE(2002) Ibogaine signals addiction genes and methamphetamine alteration of long-term potentiationAnn N Y Acad Sci965: 2846 |
182 | Criado JR, Gombart LM, Huitron-Resendiz S, Henriksen SJ(2000) Neuroadaptations in dentate gyrus function followingrepeated methamphetamine administrationSynapse37: 2163166 |
183 | Derrick BE, York AD, Martinez JLJr(2000) Increased granule cell neurogenesis in the adult dentate gyrus following mossy fiber stimulation sufficient to induce long-term potentiationBrain Res857: 1-2300307 |
184 | Chun SK, Sun W, Jung MW(2009) LTD induction suppresses LTP-induced hippocampal adult neurogenesisNeuroreport20: 1412791283 |
185 | Chun SK, Sun W, Park JJ, Jung MW(2006) Enhanced proliferation of progenitor cells following long-term potentiation induction in the rat dentate gyrusNeurobiol Learn Mem86: 3322329 |
186 | Goncalves J, Martins T, Ferreira R, Milhazes N, Borges F, Ribeiro CF(2008) Methamphetamine-induced early increase of IL-6 and TNF-alpha mRNA expression in the mouse brainAnn N Y Acad Sci1139: 103111 |
187 | Yamaguchi T, Kuraishi Y, Minami M, Nakai S, Hirai Y, Satoh M(1991) Methamphetamine-induced expression of interleukin-1 beta mRNA in the rat hypothalamusNeurosci Lett128: 19092 |
188 | Nakajima A, Yamada K, Nagai T, Uchiyama T, Miyamoto Y, Mamiya T(2004) Role of tumor necrosis factor-alpha in methamphetamine-induced drug dependence and neurotoxicityJ Neurosci24: 922122225 |
189 | Clark KH, Wiley CA, Bradberry CW(2013) Psychostimulant Abuse and Neuroinflammation: Emerging Evidence of Their InterconnectionNeurotoxicity Research23: 2174188 |
190 | Miguel-Hidalgo JJ(2009) The role of glial cells in drug abuseCurrent Drug Abuse Reviews2: 17282 |
191 | Boger HA, Middaugh LD, Granholm AC, McGinty JF(2009) Minocycline restores striatal tyrosine hydroxylase in GDNF heterozygous mice but not in methamphetamine-treated miceNeurobiol Dis33: 3459466 |
192 | Zhang L, Kitaichi K, Fujimoto Y, Nakayama H, Shimizu E, Iyo M(2006) Protective effects of minocycline on behavioral changes and neurotoxicity in mice after administration of methamphetamineProg Neuropsychopharmacol Biol Psychiatry30: 813811393 |
193 | Snider SE, Vunck SA, van den Oord EJ, Adkins DE, McClay JL, Beardsley PM(2012) The glial cell modulators, ibudilast and its amino analog, AVattenuate methamphetamine locomotor activity and its sensitization in miceEur J Pharmacol679: 1-37580 |
194 | Snider SE, Hendrick ES, Beardsley PM(2013) Glial cell modulators attenuate methamphetamine self-administration in the ratEur J Pharmacol701: 1-3124130 |
195 | Radak Z, Zhao Z, Koltai E, Ohno H, Atalay M(2013) Oxygen consumption and usage during physical exercise: The balance between oxidative stress and ROS-dependent adaptive signalingAntioxidants & Redox Signaling18: 1012081246 |
196 | Radak Z, Asano K, Inoue M, Kizaki T, Oh-Ishi S, Suzuki K(1995) Superoxide dismutase derivative reduces oxidative damage in skeletal muscle of rats during exhaustive exerciseJournal of applied physiology79: 1129135 |
197 | Radak Z, Asano K, Inoue M, Kizaki T, Oh-Ishi S, Suzuki K(1996) Superoxide dismutase derivative prevents oxidative damage in liver and kidney of rats induced by exhausting exerciseEuropean Journal of Applied Physiology and Occupational Physiology72: 3189194 |
198 | Radak Z, Kaneko T, Tahara S, Nakamoto H, Pucsok J, Sasvari M(2001) Regular exercise improves cognitive function and decreases oxidative damage in rat brainNeurochem Int38: 11723 |
199 | Ogonovszky H, Berkes I, Kumagai S, Kaneko T, Tahara S, Goto S(2005) The effects of moderate-, strenuous- and over-training on oxidative stress markers, DNA repair, and memory, in rat brainNeurochem Int46: 8635640 |
200 | Coskun S, Gonul B, Guzel NA, Balabanli B(2005) The effects of vitamin C supplementation on oxidative stress and antioxidant content in the brains of chronically exercised ratsMolecular and Cellular Biochemistry280: 1-2135138 |
201 | Suzuki M, Katamine S, Tatsumi S(1983) Exercise-induced enhancement of lipid peroxide metabolism in tissues and their transference into the brain in ratJournal of Nutritional Science and Vitaminology29: 2141151 |
202 | Somani SM, Husain K(1996) Exercise training alters kinetics of antioxidant enzymes in rat tissuesBiochemistry and Molecular Biology International38: 3587595 |
203 | Rosa EF, Takahashi S, Aboulafia J, Nouailhetas VL, Oliveira MG(2007) Oxidative stress induced by intense and exhaustive exercise impairs murine cognitive functionJ Neurophysiol98: 318201826 |
204 | Tsakiris T, Angelogianni P, Tesseromatis C, Tsakiris S, Tsopanakis C(2006) Alterations in antioxidant status, protein concentration, acetylcholinesterase, Na+, K+-ATPase, and Mg2+-ATPase activities in rat brain after forced swimmingInt J Sports Med27: 11924 |
205 | Kishi T, Hirooka Y, Katsuki M, Ogawa K, Shinohara K, Isegawa K(2012) Exercise training causes sympathoinhibition through antioxidant effect in the rostral ventrolateral medulla of hypertensive ratsClinical and Experimental Hypertension34: 4278283 |
206 | Zhang Q, Wu Y, Zhang P, Sha H, Jia J, Hu Y(2012) Exercise induces mitochondrial biogenesis after brain ischemia in ratsNeuroscience205: 1017 |
207 | Toldy A, Atalay M, Stadler K, Sasvari M, Jakus J, Jung KJ(2009) The beneficial effects of nettle supplementation and exercise on brain lesion and memory in ratThe Journal of Nutritional Biochemistry20: 12974981 |
208 | Somani SM, Ravi R, Rybak LP(1995) Effect of exercise training on antioxidant system in brain regions of ratPharmacol Biochem Behav50: 4635639 |
209 | Marosi K, Bori Z, Hart N, Sarga L, Koltai E, Radak Z(2012) Long-term exercise treatment reduces oxidative stress in the hippocampus of aging ratsNeuroscience226: 2128 |
210 | St-Pierre J, Drori S, Uldry M, Silvaggi JM, Rhee J, Jager S(2006) Suppression of reactive oxygen species and neurodegeneration by the PGC-1 transcriptional coactivatorsCell127: 2397408 |
211 | Matsui H, Ito H, Taniguchi Y, Inoue H, Takeda S, Takahashi R(2010) Proteasome inhibition in medaka brain induces the features of Parkinson’s diseaseJ Neurochem115: 1178187 |
212 | Cecarini V, Bonfili L, Cuccioloni M, Mozzicafreddo M, Rossi G, Buizza L(1822) Crosstalk between the ubiquitin-proteasome system and autophagy in a human cellular model of Alzheimer’s diseaseBiochim Biophys Acta1117411751 |
213 | Radak Z, Ihasz F, Koltai E, Goto S, Taylor AW, Boldogh I(2014) The redox-associated adaptive response of brain to physical exerciseFree Radical Research48: 18492 |
214 | Perez-Gomez A, Tasker RA(2013) Transient domoic acid excitotoxicity increases BDNF expression and activates both MEK- and PKA-dependent neurogenesis in organotypic hippocampal slicesBMC Neurosci14: 72 |
215 | Bechara RG, Lyne R, Kelly AM(2014) BDNF-stimulated intracellular signalling mechanisms underlie exercise-induced improvement in spatial memory in the male Wistar ratBehav Brain Res275: 297306 |
216 | Ji JF, Ji SJ, Sun R, Li K, Zhang Y, Zhang LY(2014) Forced running exercise attenuates hippocampal neurogenesis impairment and the neurocognitive deficits induced by whole-brain irradiation via the BDNF-mediated pathwayBiochem Biophys Res Commun443: 2646651 |
217 | Novaes Gomes FG, Fernandes J, Vannucci Campos D, Cassilhas RC, Viana GM, D’Almeida V(2014) The beneficial effects of strength exercise on hippocampal cell proliferation and apoptotic signaling is impaired by anabolic androgenic steroidsPsychoneuroendocrinology50: 106117 |
218 | Ploughman M, Austin MW, Glynn L, Corbett D(2014) The effects of poststroke aerobic exercise on neuroplasticity: A systematic review of animal and clinical studiesTranslational stroke research |
219 | Murphy DD, Segal M(1997) Morphological plasticity of dendritic spines in central neurons is mediated by activation of cAMP response element binding proteinProc Natl Acad Sci U S A94: 414821487 |
220 | Holmes MM, Galea LA, Mistlberger RE, Kempermann G(2004) Adult hippocampal neurogenesis and voluntary running activity: Circadian and dose-dependent effectsJ Neurosci Res76: 2216222 |
221 | van Praag H, Shubert T, Zhao C, Gage FH(2005) Exercise enhances learning and hippocampal neurogenesis in aged miceJ Neurosci25: 3886808685 |
222 | Noble M, Mayer-Proschel M, Proschel C(2005) Redox regulation of precursor cell function: Insights and paradoxesAntioxidants & Redox Signaling7: 11-1214561467 |
223 | Tonchev AB(2011) Brain ischemia, neurogenesis, and neurotrophic receptor expression in primatesArchives Italiennes de Biologie149: 2225231 |
224 | Cheng A, Coksaygan T, Tang H, Khatri R, Balice-Gordon RJ, Rao MS(2007) Truncated tyrosine kinase B brain-derived neurotrophic factor receptor directs cortical neural stem cells to a glial cell fate by a novel signaling mechanismJ Neurochem100: 615151530 |
225 | Somkuwar SS, Staples MC, Galinato MH, Fannon MJ, Mandyam CD(2014) Role of NG2 expressing cells in addiction: A new approach for an old problemFrontiers in Pharmacology5: 279 |
226 | Balodis IM, Molina ND, Kober H, Worhunsky PD, White MA, Rajita S(2013) Divergent neural substrates of inhibitory control in binge eating disorder relative to other manifestations of obesityObesity (Silver Spring)21: 2367377 |
227 | Dalley JW, Cardinal RN, Robbins TW(2004) Prefrontal executive and cognitive functions in rodents: Neural and neurochemical substratesNeurosci Biobehav Rev28: 7771784 |
228 | Pinilla FG(2006) The impact of diet and exercise on brain plasticity and diseaseNutr Health18: 3277284 |
229 | Yu F, Kolanowski AM, Strumpf NE, Eslinger PJ(2006) Improving cognition and function through exercise intervention in Alzheimer’s diseaseJ Nurs Scholarsh38: 4358365 |
230 | O’Dell SJ, Galvez BA, Ball AJ, Marshall JF(2012) Running wheel exercise ameliorates methamphetamine-induced damage to dopamine and serotonin terminalsSynapse66: 17180 |
231 | O’Dell SJ, Marshall JF(2014) Running wheel exercise before a binge regimen of methamphetamine does not protect against striatal dopaminergic damageSynapse68: 9419425 |
232 | Mathes WF, Nehrenberg DL, Gordon R, Hua K, Garland TJr, Pomp D(2010) Dopaminergic dysregulation in mice selectively bred for excessive exercise or obesityBehav Brain Res210: 2155163 |
233 | Waters RP, Pringle RB, Forster GL, Renner KJ, Malisch JL, Garland TJr(1508) Selection for increased voluntary wheel-running affects behavior and brain monoamines in miceBrain Res922 |
234 | Rhodes JS, Garland TJr, Gammie SC(2003) Patterns of brain activity associated with variation in voluntary wheel-running behaviorBehav Neurosci117: 612431256 |
235 | Johnson RA, Rhodes JS, Jeffrey SL, Garland TJr, Mitchell GS(2003) Hippocampal brain-derived neurotrophic factor but not neurotrophin-3 increases more in mice selected for increased voluntary wheel runningNeuroscience121: 117 |
236 | Hamer M, Endrighi R, Poole L(2012) Physical activity, stress reduction, and mood: Insight into immunological mechanismsMethods Mol Biol934: 89102 |
237 | Bernardi C, Tramontina AC, Nardin P, Biasibetti R, Costa AP, Vizueti AF(2013) Treadmill exercise induces hippocampal astroglial alterations in ratsNeural Plast709732 |
238 | Nielsen DA, Utrankar A, Reyes JA, Simons DD, Kosten TR(2012) Epigenetics of drug abuse: Predisposition or responsePharmacogenomics13: 1011491160 |
239 | Nestler EJ(2014) Epigenetic mechanisms of drug addictionNeuropharmacology76 Pt B: 259268 |
240 | Aguilar-Valles A, Vaissiere T, Griggs EM, Mikaelsson MA, Takacs IF, Young EJ(2014) Methamphetamine-associated memory is regulated by a writer and an eraser of permissive histone methylationBiol Psychiatry76: 15765 |
241 | Cadet JL, Jayanthi S(2013) Epigenetics of methamphetamine-induced changes in glutamate functionNeuropsychopharmacology38: 1248249 |
242 | Schmidt HD, McGinty JF, West AE, Sadri-Vakili G(2013) Epigenetics and psychostimulant addictionCold Spring Harbor perspectives in medicine3: 3a012047 |
243 | Zoladz JA, Pilc A(2010) The effect of physical activity on the brain derived neurotrophic factor: From animal to human studiesJournal of Physiology and Pharmacology: An Official Journal of the Polish Physiological Society61: 5533541 |
244 | Gomez-Pinilla F, Zhuang Y, Feng J, Ying Z, Fan G(2011) Exercise impacts brain-derived neurotrophic factor plasticity by engaging mechanisms of epigenetic regulationEur JNeurosci33: 3383390 |
245 | Foley TE, Fleshner M(2008) Neuroplasticity of dopamine circuits after exercise: Implications for central fatigueNeuromolecular Med10: 26780 |
246 | Loweth JA, Tseng KY, Wolf ME(2013) Using metabotropic glutamate receptors to modulate cocaine’s synaptic and behavioral effects: mGluR1 finds a nicheCurr OpinNeurobiol23: 4500506 |
247 | Pickens CL, Airavaara M, Theberge F, Fanous S, Hope BT, Shaham Y(2011) Neurobiology of the incubation of drug cravingTrends Neurosci34: 8411420 |
248 | Rothman RB, Baumann MH(2003) Monoamine transporters and psychostimulant drugsEur J Pharmacol479: 1-32340 |
249 | Kish SJ(2008) Pharmacologic mechanisms of crystal methCMAJ: Canadian Medical Association Journal = Journal de l’Association Medicale Canadienne178: 1316791682 |
250 | Chen HI, Kuo YM, Liao CH, Jen CJ, Huang AM, Cherng CG(2008) Long-term compulsive exercisereduces the rewarding efficacy of 3,4-methylenedioxy-methamphetamineBehav Brain Res187: 1185189 |
251 | Marques E, Vasconcelos F, Rolo MR, Pereira FC, Silva AP, Macedo TR(2008) Influence of chronic exercise on the amphetamine-induced dopamine release and neurodegeneration in the striatum of the ratAnn N Y Acad Sci1139: 222231 |
252 | Sutoo DE, Akiyama K(1996) The mechanism by which exercise modifies brain functionPhysiol Behav60: 1177181 |
253 | Droste SK, Schweizer MC, Ulbricht S, Reul JM(2006) Long-term voluntary exercise and the mouse hypothalamic-pituitary-adrenocortical axis: Impact of concurrent treatment with the antidepressant drug tianeptineJ Neuroendocrinol18: 12915925 |
254 | Kolb EM, Rezende EL, Holness L, Radtke A, Lee SK, Obenaus A(2013) Mice selectively bred for high voluntary wheel running have larger midbrains: Support for the mosaic model of brain evolutionJ Exp Biol216: Pt 3515523 |
255 | O’Neil ML, Kuczenski R, Segal DS, Cho AK, Lacan G, Melega WP(2006) Escalating dose pretreatment induces pharmacodynamic and not pharmacokinetic tolerance to a subsequent high-dose methamphetamine bingeSynapse60: 6465473 |
256 | Zhang Y, Loonam TM, Noailles PA, Angulo JA(2001) Comparison of cocaine- and methamphetamine-evoked dopamine and glutamate overflow in somatodendritic and terminal field regions of the rat brain during acute, chronic, and early withdrawal conditionsAnn N Y Acad Sci937: 93120 |
257 | Real CC, Ferreira AF, Hernandes MS, Britto LR, Pires RS(1363) Exercise-induced plasticity of AMPA-type glutamate receptor subunits in the rat brainBrain Res6371 |
258 | Biedermann S, Fuss J, Zheng L, Sartorius A, Falfan-Melgoza C, Demirakca T(2012) In vivo voxel based morphometry: Detection of increased hippocampal volume and decreased glutamate levels in exercising miceNeuroimage61: 412061212 |
259 | Guezennec CY, Abdelmalki A, Serrurier B, Merino D, Bigard X, Berthelot M(1998) Effects of prolonged exercise on brain ammonia and amino acidsInt J Sports Med19: 5323327 |
260 | Crawford JT, Roberts DC, Beveridge TJ(2013) The group II metabotropic glutamate receptor agonist, LY68, decreases methamphetamine self-administration in ratsDrug Alcohol Depend132: 3414419 |
261 | Schwendt M, Reichel CM, See RE(2012) Extinction-dependent alterations in corticostriatal mGluR2/3 and mGluR7 receptors following chronic methamphetamine self-administration in ratsPLoS ONE7: 3e34299 |
262 | Kufahl PR, Watterson LR, Nemirovsky NE, Hood LE, Villa A, Halstengard C(2013) Attenuation of methamphetamine seeking by the mGluR2/3 agonist LY68 in rats with histories of restricted and escalated self-administrationNeuropharmacology66: 290301 |
263 | Nestler EJ(2001) Molecular neurobiology of addictionAm J Addict10: 3201217 |
264 | Werme M, Thoren P, Olson L, Brene S(2000) Running and cocaine both upregulate dynorphin mRNA in medial caudate putamenEur J Neurosci12: 829672974 |
265 | Werme M, Messer C, Olson L, Gilden L, Thoren P, Nestler EJ(2002) Delta FosB regulates wheel runningJ Neurosci22: 1881338138 |
266 | Smith MA, Yancey DL(2003) Sensitivity to the effects of opioids in rats with free access to exercise wheels: Mu-opioid tolerance and physical dependencePsychopharmacology (Berl)168: 4426434 |
267 | Iijima M, Koike H, Chaki S(2013) Effect of an mGlu2/3 receptor antagonist on depressive behavior induced by withdrawal from chronic treatment with methamphetamineBehav Brain Res246: 2428 |
268 | McDaid J, Graham MP, Napier TC(2006) Methamphetamine-induced sensitization differentially alters pCREB and DeltaFosB throughout the limbic circuit of the mammalian brainMol Pharmacol70: 620642074 |
269 | Wang JQ, McGinty JF(1995) Dose-dependent alteration in zif/268 and preprodynorphin mRNA expression induced by amphetamine or methamphetamine in rat forebrainJ Pharmacol Exp Ther273: 2909917 |
Figures and Tables
Fig.1
Neurobiological Overlap of Methamphetamine and Exercise in the Adult Rodent Brain: A schematic for the overarching effects of methamphetamine and exercise on the reward, reinforcement and motivational centers of the adult rodent brain, particularly the prefrontal cortex (highlighted in green), the hippocampus (highlighted in blue), and striatum (highlighted in pink).
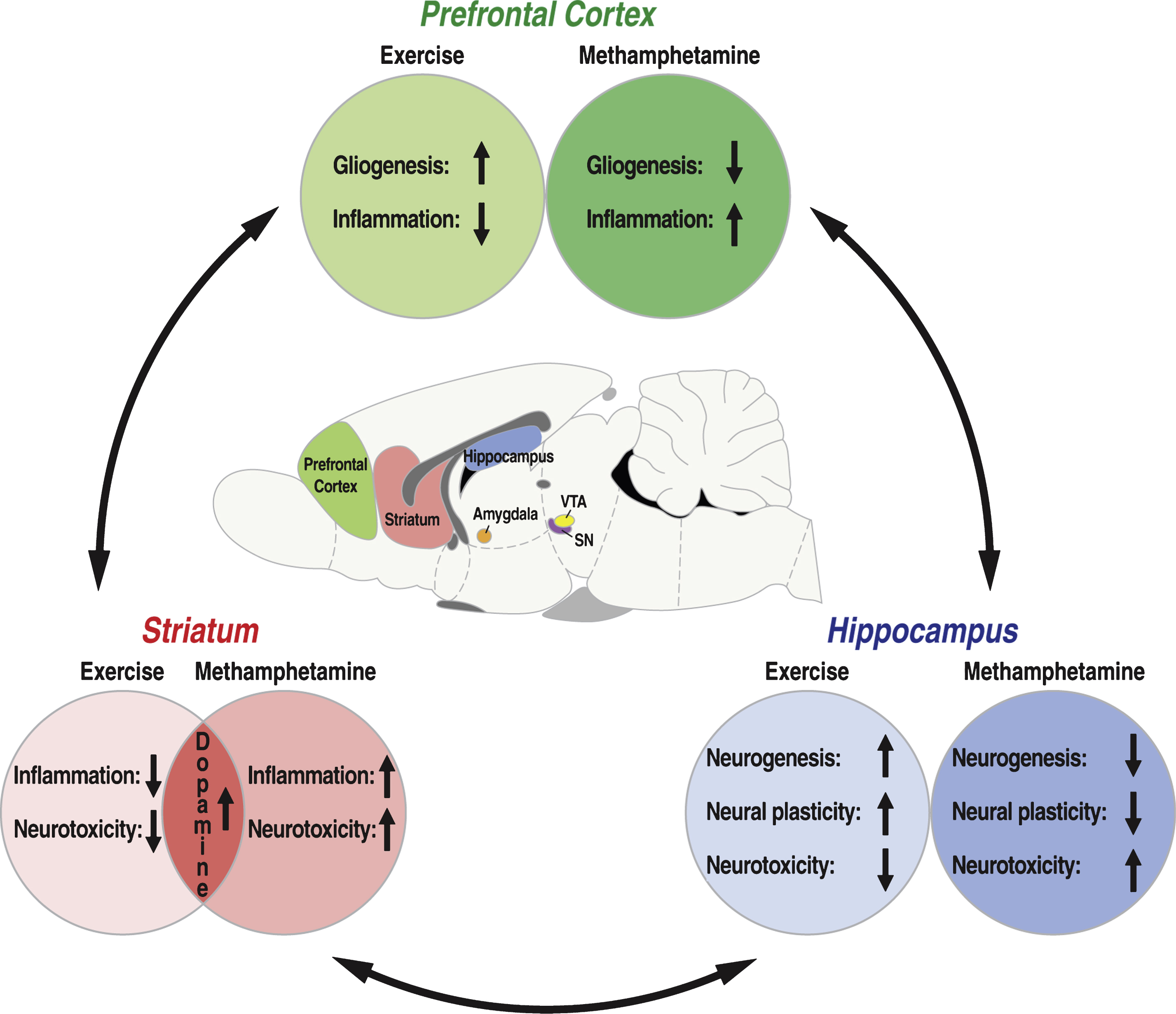
Table 1
Exercise and methamphetamine alter neurotransmission in the reward and reinforcement centers of the brain. This contributes to the value of exercise as a treatment for drug addiction (for review, [7, 245]). Of interest are dopaminergic and glutamatergic systems. The acute reinforcing actions of stimulants, like methamphetamine, has been attributed to the mesolimbic dopamine system that initiates in ventral tegmental area and terminates in the nucleus accumbens [2]. The transition of drug abuse behavior to prolonged intoxication and preoccupation/anticipation stage is accompanied by adaptations of the glutamatergic system [246, 247], which, in part, contribute to negative affect (example, depression and anxiety) during drug withdrawal [2]
Exercise | Methamphetamine and effect of exercise |
Acute exercise activates mesolimbic dopamine pathway [57]. | Methamphetamine increases extracellular dopamine in the brain by increasing release and reducing uptake [248, 249]. |
Exercise blunts the dopaminergic response to initial methamphetamine experience [250, 251]. | |
Chronic running as well as predisposition for increased running induces dopaminergic adaptations [57, 252–254]. | Chronic methamphetamine also produces neuroadaptations that lead to tolerance and hypofunctionalilty in the mesocorticolimbic dopamine pathway [255, 256]. This contributes to compensatory escalation of methamphetamine intake, and may further enhance relapse vulnerability. |
A) Some of these adaptations may be beneficial. | A) Chronic exercise experience and continued access to exercise attenuated escalation of and relapse to methamphetamine self-administration [58, 59]. |
B) Some may be detrimental and contribute to escalation of exercise, particularly when exercise is withdrawn [57, 60]. | B) Chronic exercise sensitized the reward system for other drug reinforcers, particularly when access to exercise is withdrawn [58, 60] |
Increase expression of group II metabotropic glutamate receptors (mGluR2/3) in striatum, and dampen glutamate release and signaling in the striatum and the hippocampus [257–259] | Dysregulation of glutamatergic signaling, and internalization-mediated decrease of mGluR2/3 in the prefrontal cortex, nucleus accumbens and dorsal striatum is evidenced in methamphetamine escalation [260–262]. Activation of mGluR2/3 reduces methamphetamine seeking both before and after development of escalation [260] |
Molecular markers associated with the negative affect and dependence, like increased ΔFosB and dynorphin were also found to be upregulated by exercise [263–265] withdrawal from exercise may result in the manifestation of negative affect [8, 62, 266]. | Activation of mGluR2/3 in the nucleus accumbens during withdrawal from chronic methamphetamine administration is associated with depressive behavior [267]. Negative affect markers, ΔFosB and dynorphin, were upregulated by chronic methamphetamine [263, 268, 269], all of which may increase relapse risk. |