SH3BP5-AS1/IGF2BP2/VDAC2 Axis Promotes the Apoptosis and Ferroptosis of Bladder Cancer Cells
Abstract
BACKGROUND:
Bladder cancer (BC) is the most common malignant tumor in the urinary system with a high incidence, imposing a burden on the healthcare system worldwide. The participation of long non-coding RNAs (lncRNAs) in BC has attracted increasing attention.
OBJECTIVE:
The aim in the current study was to explore the potential mechanism involving SH3BP5-AS1 in modulating BC cell proliferation, apoptosis and ferroptosis.
METHODS:
qPCR and WB analysis measured the expression of RNAs and proteins. Functional and mechanism experiments were performed to investigate RNA impacts on cell proliferation, apoptosis and ferroptosis, and explore the correlation between RNA and protein expression.
RESULTS:
SH3BP5-AS1 was down-regulated in BC cells, and SH3BP5-AS1 overexpression could inhibit BC cell proliferation but facilitate the cell apoptosis. SH3BP5-AS1 was also found to facilitate the ferroptosis of BC cells. Additionally, SH3BP5-AS1 was confirmed to recruit IGF2BP2 to regulate VDAC2 expression in the m6A-dependent manner. VDAC2 was detected to be down-regulated in BC cells and was verified to inhibit BC cell growth. Moreover, it was indicated from rescue assays that SH3BP5-AS1 could modulate VDAC2 expression to promote the ferroptosis of BC cells.
CONCLUSION:
SH3BP5-AS1 could affect BC cell proliferation, apoptosis and ferroptosis via IGF2BP2/VDAC2, providing a novel molecular perspective for understanding BC.
INTRODUCTION
Bladder cancer (BC) is one of the most prevalent urinary malignancies, severely affecting the quality of human life due to its mortality and recurrence [1, 2]. Classifying patients into low-, intermediate-, and high-risk groups is of crucial importance to the recommendation of treatment, including immediate chemotherapy instillation, intravesical immunotherapy and radical cystectomy [3]. Accumulating studies have made important contributions to BC treatment due to the discovery of novel diagnosis biomarkers, but the mortality displays less marked changes despite advancements in BC detection and treatment, and the five-year overall survival remains unsatisfying [4]. Therefore, further investigation into the molecular mechanisms still deserves much attention for the discovery of potential diagnostic markers.
The participation of long non-coding RNAs (lncRNAs) in modulating the malignant behaviors of tumor cells has been much discussed, and significant functions of lncRNAs have also been validated in such aspects as the inactivation of X-chromosomal, remodeling of chromatin and transcriptional repression [5]. Functions of lncRNAs in BC have also attracted much attention in recent years, and advancements have been achieved in the analysis of molecular mechanisms essential to BC cell growth and tumor progression. Specifically, lncRNA RMRP could function as a miR-206 sponge to affect the proliferation, migration and invasion of BC cells, and more importantly, the close relation between RMRP expression levels and lymph node metastasis in BC has been fathomed out [6]. LncRNA CASC11, based on recent literature, is also testified to impact BC cell proliferation via miRNA-150 [7]. Moreover, exosomal PTENP1 is validated to exert inhibitory impacts on the malignant behaviors of BC cells via the regulation on PTEN/microRNA-17 [8]. LncRNA SH3BP5-AS1 is up-regulated in cells extracted from recurrent neuroblastoma tissues and displays differential expressions in neuroblastoma patients, and based on further analysis, SH3BP5-AS1 could be considered as a significant factor for the clinical prediction of neuroblastoma outcomes [9]. According to a recently published report, SH3BP5-AS1 is down-regulated in BC tissues when compared with paracancerous tissues [10]. The specific functions and regulatory mechanism of SH3BP5-AS1 in BC require further investigation.
Ferroptosis is a novel, non-apoptotic type of cell death in an iron-dependent pattern and characterized with the accumulation of lipid peroxidation products and lethal reactive oxygen species (ROS) derived from iron metabolism [11–13]. The significant involvement of ferroptosis in the occurrence and progression of various malignancies has been somewhat analyzed. To be specific, ferroptosis is closely associated with intestinal ischemia/reperfusion injury [14] and the immune system is also verified to partially function through ferroptosis for the prevention of tumorigenesis [15]. Additionally, the potential correlation between lncRNAs and ferroptosis in cancers has also been unveiled. For instance, cytosolic P53RRA interacts with G3BP1 for nuclear p53 retention, thus contributing to cell-cycle arrest and ferroptosis [16]. ZFAS1 silence is confirmed to attenuate the ferroptosis and pulmonary fibrosis progression via modulating miR-150-5p/SLC38A1 [17]. Herein, we also tried to figure out whether there was a correlation between SH3BP5-AS1 and ferroptosis in BC.
In the current study, the main aim was to probe into SH3BP5-AS1 impacts on proliferation, apoptosis and ferroptosis of BC cells, and the corresponding modulation mechanism was also investigated.
MATERIALS AND METHODS
Cell culture
Immortalized human urothelial cell line (SV-HUC-1) and human BC cell lines (T24, J82, 5637, HT1376) were procured from American Type Culture Collection (ATCC). The procured cells were incubated in RPMI 1640 medium (61870127, Gibco, USA) supplemented with 10% fetal bovine serum (FBS; 10270-106, Gibco) and 100 U/ml penicillin-streptomycin solution in a humidified incubator with 5% CO2 at 37°C, respectively.
Quantitative real time PCR (qPCR)
Total RNA was extracted with Trizol (9108-1, Thermo Fisher, USA) according to the manufacturer’s protocol. cDNA was synthesized from total RNA (1μg) with PrimeScript™ RT Master Mix. qPCR was carried out with ChamQ Universal SYBR qPCR Master Mix (Q711-02, Vazyme Biotech, Nanjing, China). The results were calculated and presented as 2–ΔΔCt. GAPDH was regarded as the internal control. The experiment was repeated for three times.
Cell transfection
For overexpressing SH3BP5-AS1, IGF2BP2 and VDAC2, pcDNA3.1 vectors were obtained to construct pcDNA3.1-SH3BP5-AS1, pcDNA3.1-IGF2BP2 and pcDNA3.1-VDAC2, respectively. Short hairpin RNAs (shRNAs) targeting SH3BP5-AS1, IGF2BP2 and VDAC2 were purchased for the silence of SH3BP5-AS1, IGF2BP2 and VDAC2 by constructing sh-SH3BP5-AS1, sh-IGF2BP2 and sh-VDAC2. After the indicated plasmids were transfected into cells with Lipofectamine 2000, qPCR was applied to select the cells with stable transfection.
Cell counting kit-8 (CCK-8)
The Cell Counting Kit-8 (M4839, ABMOLE, USA) was obtained to detect cell viability, with the manufacturer’s instructions strictly followed. Cells were seeded onto 96-well flat-bottomed plate (1,000 cells per well) and incubated at 37°C for 24 h, followed by the transfection of corresponding plasmids. CCK-8 solution was added to each well and the absorbance was determined at 450 nm by using a microplate reader (51119770DP, Thermo Fisher). The data was collected for 3 days. The experiment was repeated for three times.
Flow cytometry assay
Cell apoptosis was detected with Flow Cytometer (DxFLEX, Thermo Fisher) and Annexin V/PI staining kit according to the standard protocol. After digestion and re-suspension in the binding buffer, cells (4×106) in 6-well plates were double-stained with Annexin V/PI staining kit at room temperature. Afterwards, the apoptotic cells were analyzed with flow cytometer. The experiment was repeated for three times.
Iron detection assay
The iron assay kit (ab83366, Abcam, Cambridge, MA, UK) was procured for measuring Fe2 + in cells. Transfected cells were seeded onto 96-well plates (3,000 cells per well). Subsequent to the removal of insoluble materials, ferric iron was dissociated into solution by ferric carrier proteins in an acid buffer environment. After the iron was reduced to ferrous form and reacted with Ferene S to form a stable colored complex, the ferrous iron was measured, followed by analysis with a microplate reader. The experiment was repeated for three times.
Measurement of lipid reactive oxygen species (ROS)
Lipid ROS measurement was employed as previously described [18]. Cells (4×105/ml) were treated with DSF/Cu (1μM/1μM) for 6 h and washed with PBS (C10010500BT, Gibco). Then, cells were incubated with 10μM DCFH-DA in serum-free medium at 37°C for 30 min in a dark place. After PBS washes, lipid ROS levels were analyzed on a flow cytometer using a 519 nm filter for DCFH-DA fluorescein detection. Data were collected from at least 10,000 cells per sample. The experiment was repeated for three times.
Fluorescent in situ hybridization (FISH)
FISH assay was completed with the help of FISH probe kit (C10910, RIBOBIO, Guangzhou, China). Fixed cells (8,000–10,000 cells per well in 24-well plates) were gathered for the incubation in the hybridization buffer with SH3BP5-AS1 FISH probe, and the negative control probes (without specific labeling) were also utilized. Subsequently, DAPI solution was supplemented for counterstaining the nuclei and then the stained cells were observed and analyzed with laser scanning confocal microscope (Smart zoom 5, ZEISS). The experiment was repeated for three times.
Luciferase reporter assay
For the detection of methylation site on VDAC2, VDAC2 with wide-type m6A, VDAC2 m6A with mutated site 1206 or mutated site 1199 was respectively sub-cloned into pGL3 vectors to construct pGL3-VDAC2 (m6A-Wt), pGL3-VDAC2 (m6A-1206 Mut) or pGL3-VDAC2 (m6A-1199 Mut). The obtained plasmids were transfected into cells (1.2×105 cells per well in 24-well plates) with co-transfection of pcDNA3.1 or pcDNA3.1-IGF2BP2. Dual luciferase reporter kit (RG027, Beyotime Biotechnology, Shanghai, China) was applied to detect the luciferase activity. The experiment was repeated for three times.
Western blot (WB) assay
Cells were lysed in RIPA buffer for the extraction of proteins. Total cell proteins were separated by 10% SDS-PAGE before being transferred to polyvinylidene difluoride (PVDF) membranes. The proteins on PVDF membranes were then probed with Anti-β-actin (ab8226, Abcam), Anti-VDAC2 (ab37985, Abcam), and Anti-IGF2BP2 (ab128175, Abcam). The secondary antibodies conjugated with HRP (ab131368, Abcam) were added for subsequent 2-h cultivation with samples. Finally, the proteins were measured via enhanced chemiluminescence (ECL) detection system. 30μg proteins were loaded onto each lane. The experiment was repeated for three times.
RNA pulldown
To verify the relation among different factors, sense or anti-sense SH3BP5-AS1, sense or antisense VDAC2, and VDAC21206M sense were respectively procured. Cells (2×107) were lysed in the lysis buffer preliminarily. To verify the relation between SH3BP5-AS1/VDAC2 and IGF2BP2, sense or antisense SH3BP5-AS1/VDAC2 was added into cell lysates. In addition, sense or antisense VDAC2 and VDAC21206M sense was used to pull down IGF2BP2 in order to confirm the methylation site. Pierce™ Streptavidin Magnetic Beads (88816, Thermo Fisher) were applied to pull down the biotinylated RNA-protein duplexes. The purified protein was subjected to WB analysis. The experiment was repeated for three times.
RNA binding protein immunoprecipitation (RIP)
RIP assay in this study was completed with Imprint® RNA Immunoprecipitation Kit (RIP-12RXN, Sigma-Aldrich, USA). Firstly, 2×107 cells were centrifuged and re-suspended in RIP lysis buffer after being washed with PBS. Cell lysates were co-cultured with Anti-IgG (ab133470, Abcam), Anti-SRSF1 (sc-33652, Santa Cruz, CA, USA), Anti-U2AF2 (ab37530, Abcam), Anti-HNRNPA1 (ab208027, Abcam) and Anti-IGF2BP2 (ab128175, Abcam), which were pre-conjugated with magnetic beads. The precipitated RNAs in the complexes were isolated and assessed by qPCR. The experiment was repeated for three times.
Statistical analysis
All statistical analyses were performed with Statistical Product and Service Solutions (SPSS) 20.0 software. GraphPad Prism 7.0 software was used to draw graphs. The data were expressed as the mean±SD, and each assay was carried out for at least three times. Multiple comparisons were performed using Student’s t-test, one-way analysis of variance (ANOVA) and two-way ANOVA. P < 0.05 was regarded as statistically significant.
RESULTS
SH3BP5-AS1 impedes BC cell proliferation but facilitates BC cell apoptosis
In accordance to the bioinformatics analysis conducted by Cao et al., SH3BP5-AS1, a member of immune-related lncRNAs, was down-regulated in BC tissues and cells relative to paracancerous tissues and normal bladder epithelium cells [10]. In the current study, before investigation into SH3BP5-AS1 influences on BC cell behaviors, qPCR analysis was first conducted to determine the expression level of SH3BP5-AS1 in different cell lines. As we expected, compared with immortalized human urothelial cell line (SV-HUC-1), SH3BP5-AS1 displayed a lower expression in BC cell lines, particularly in T24 and J82 cells, and the two cell lines were selected for implementing the subsequent experiments (Fig. 1A). Then, pcDNA3.1-SH3BP5-AS1 was transfected into T24 and J82 cells to overexpress SH3BP5-AS1 (Fig. 1B). CCK-8 assay corroborated that SH3BP5-AS1 up-regulation led to the decreased cell proliferation (Fig. 1C), while flow cytometry assay substantiated that BC cell apoptosis was facilitated by pcDNA3.1-SH3BP5-AS1 transfection (Fig. 1D). Next, we evaluated the subcellular distribution of SH3BP5-AS1 through FISH assay, and it was manifested that SH3BP5-AS1 existed both in cell nucleus and cytoplasm (Fig. 1E). To conclude, SH3BP5-AS1 is ascertained to impede cell proliferation and facilitate cell apoptosis in BC.
Fig. 1
SH3BP5-AS1 impedes BC cell proliferation but facilitates BC cell apoptosis. (A) qPCR analysis was done for calculating the expression level of SH3BP5-AS1 in immortalized human urothelial cell line (SV-HUC-1) and human BC cell lines (T24, J82, 5637, HT1376). (B) SH3BP5-AS1 expression was detected in pcDNA3.1-SH3BP5-AS1-transfected cells via qPCR. (C) The viability of pcDNA3.1-SH3BP5-AS1-transfected cells was evaluated via CCK-8 assay. (D) The apoptosis of pcDNA3.1-SH3BP5-AS1-transfected cells was analyzed via flow cytometry assay. (E) FISH assay was done to determine the cellular distribution of SH3BP5-AS1 in T24 and J82 cells. *p < 0.05, **p < 0.01.
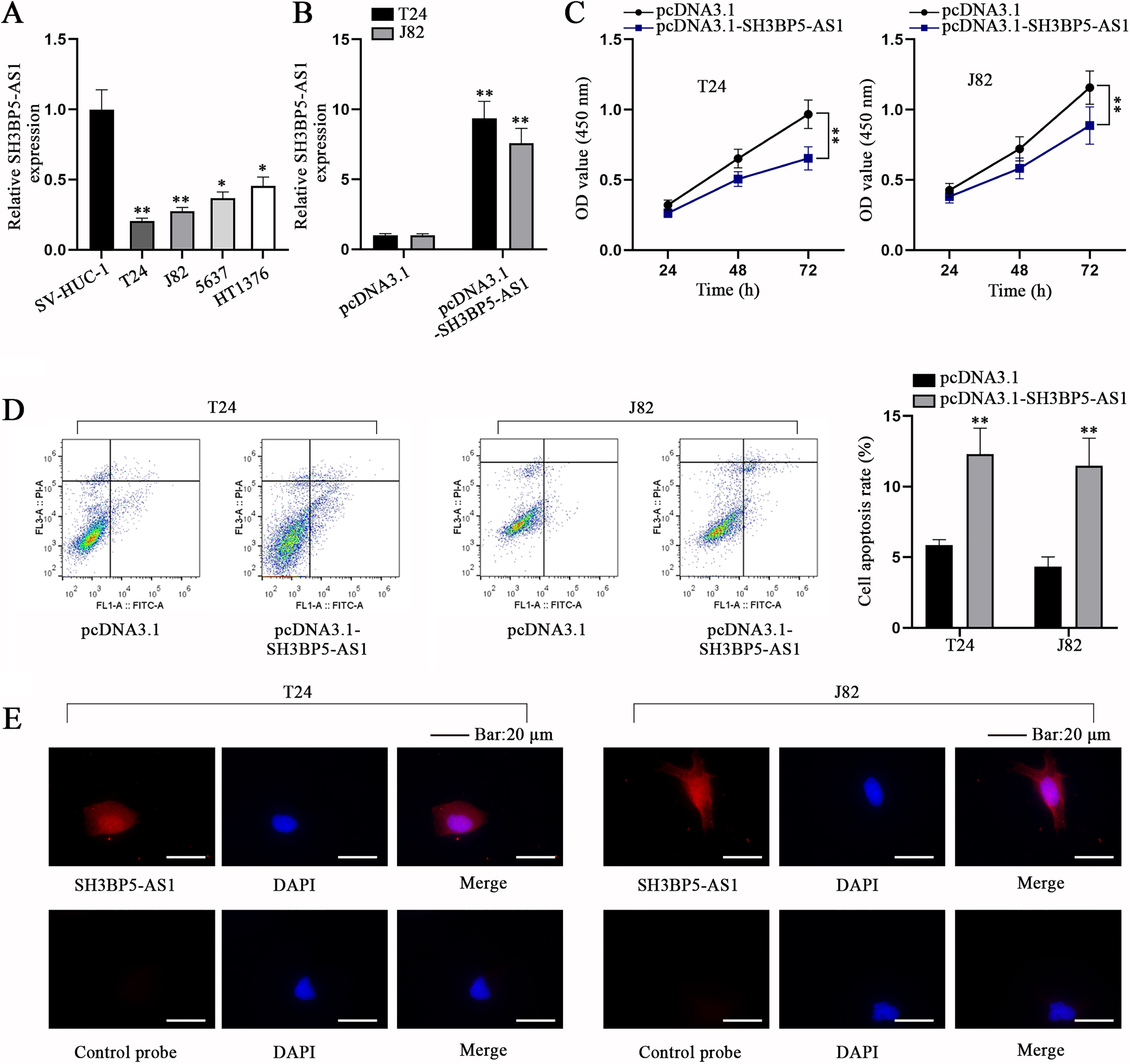
SH3BP5-AS1 facilitates BC cell ferroptosis
LncRNAs might function by interacting with RNA binding proteins (RBPs) in cancer cells [19]. Therefore, we wondered whether there existed potential interaction between SH3BP5-AS1 and a certain RBP in BC cells. According to starBase prediction (https://starbase.sysu.edu.cn/index.php) under the conditions of CLIP-Data > = 5 and pan-Cancer > = 10, the top 4 RBPs with highest ClusterNum (SRSF1, U2AF2, HNRNPA1 and IGF2BP2) were screened out (Fig. 2A). The illustration of ferroptosis mechanism acquired from KEGG website (https://www.kegg.jp/) was displayed (Fig. 2B). Intriguingly, 4 of the above-mentioned RBPs were also predicted to bind with various key ferroptosis genes on starBase (Fig. 2C; Supplementary file). The crucial association between ferroptosis and lncRNAs has been somewhat raveled out [20], and the previous study has also unveiled the role of RBPs in modulating cell ferroptosis in cancers [21]. Hence, we speculated that SH3BP5-AS1 could interact with RBPs to modulate specific ferroptosis genes, thus affecting BC cell ferroptosis. Ferroptosis is usually accompanied by a large amount of iron accumulation and lipid ROS [22]. To validate our speculation, Fe2 + in BC cells before and after SH3BP5-AS1 overexpression was detected. According to the experimental results, we found that Fe2 + content was largely increased by SH3BP5-AS1 augment (Fig. 2D). Lipid ROS level was also detected via flow cytometry, and it was revealed that SH3BP5-AS1 overexpression could induce a higher lipid ROS level (Fig. 2E). Herein, it is confirmed that SH3BP5-AS1 can affect BC cell ferroptosis.
Fig. 2
SH3BP5-AS1 facilitates BC cell ferroptosis. (A) Bioinformatics tool (starBase) was applied to predict the RBPs potentially binding to SH3BP5-AS1 under the indicated conditions (CLIP Data> = 5, pan-Cancer> = 10). (B) The ferroptosis pathway map obtained from KEGG was demonstrated. (C) The four candidate RBPs (U2AF2, HNRNPA1, SRSF1 and IGF2BP2) were predicted to potentially bind with key genes of ferroptosis on starBase. (D) The ferrous iron content in T24 and J82 cells with overexpressed SH3BP5-AS1 was measured with a microplate reader. (E) Flow cytometer was applied to detect lipid ROS in BC cells with SH3BP5-AS1 up-regulation. **p < 0.01.
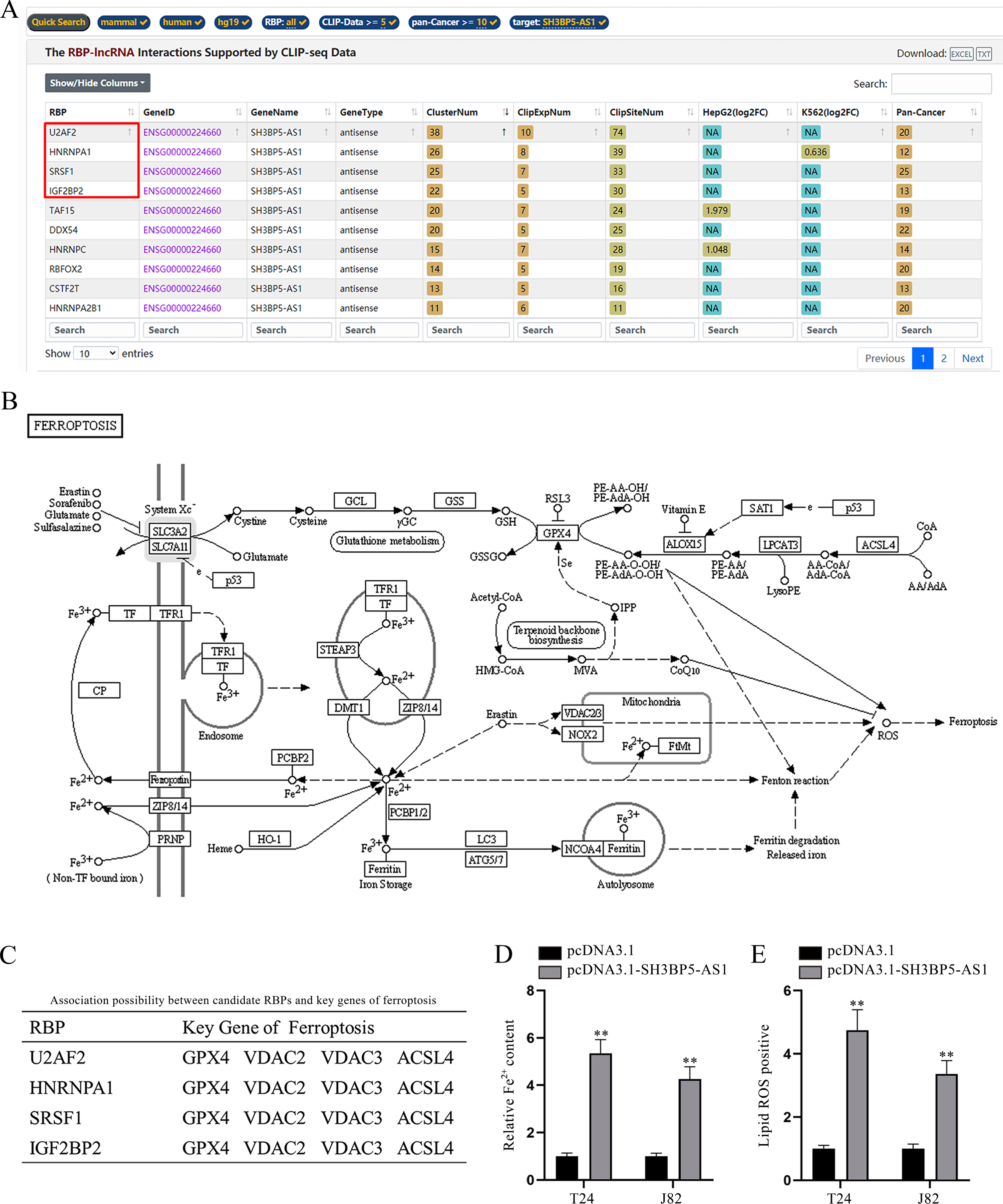
SH3BP5-AS1 recruits IGF2BP2 to positively modulate VDAC2
To further explore the underlying regulatory mechanism of SH3BP5-AS1, we conducted RIP assay to precipitate SH3BP5-AS1 with antibodies against the predicted RBPs, and Anti-IgG was used as the negative control. By analyzing SH3BP5-AS1 enrichment, it could be ascertained that IGF2BP2 was the RBP binding to SH3BP5-AS1 in T24 and J82 cells, as only IGF2BP2 antibody was able to significantly precipitate SH3BP5-AS1 (Fig. 3A). It had already been displayed in Fig. 2C that IGF2BP2 was possibly associated with some ferroptosis genes including GPX4, VDAC2, VDAC3 and ACSL4. VDAC2 was screened out as the downstream key ferroptosis gene via RIP, as VDAC2 enrichment in IGF2BP2 antibody was substantial (Fig. 3B). In RNA pulldown assay, IGF2BP2 was pulled down by both biotinylated SH3BP5-AS1 sense and VDAC2 sense, which further proved the combination between IGF2BP2 and SH3BP5-AS1 or between IGF2BP2 and VDAC2 (Fig. 3C-D). Then, we conducted WB analysis to detect IGF2BP2 and VDAC2 expression in cells with SH3BP5-AS1 overexpression, and found that SH3BP5-AS1 augment could only enhance the protein level of VDAC2, while that of IGF2BP2 remained almost unchanged (Fig. 3E). Subsequently, IGF2BP2 was silenced in T24 and J82 cells, and sh-IGF2BP2-1 was chosen in the following experiments due to the highest knockdown efficiency (Fig. 3F). Hereafter, sh-IGF2BP2-1 was named as sh-IGF2BP2. The results of qPCR and WB analysis indicated that VDAC2 mRNA and protein levels were decreased after cells were transfected with sh-IGF2BP2 (Fig. 3G). Furthermore, as depicted in Fig. 3H, VDAC2 level precipitated in Anti-IGF2BP2 was detected to be higher in pcDNA3.1-SH3BP5-AS1-transfected cells than in pcDNA3.1-transfected cells. Additionally, we conducted qPCR and WB analyses to detect the influence of SH3BP5-AS1/IGF2BP2 on VDAC2 expression, and found that the decline in VDAC2 mRNA and protein levels, resulting from IGF2BP2 depletion, could not be recovered by SH3BP5-AS1 overexpression (Fig. 3I). In all, SH3BP5-AS1 recruits IGF2BP2 to enhance VDAC2 expression in BC cells.
Fig. 3
SH3BP5-AS1 recruits IGF2BP2 to positively regulate VDAC2. (A) In RIP assay, the enrichment of SH3BP5-AS1 in Anti-U2AF2, Anti-HNRNPA1, Anti-IGF2BP2 and Anti-SRSF1 was calculated by qPCR. (B) The possible binding between SH3BP5-AS1 and four RBPs was evaluated by RNA pulldown assay. (C-D) IGF2BP2 level pulled down by SH3BP5-AS1/VDAC2 sense was detected via WB. (E) WB was done to measure IGF2BP2 and VDAC2 protein levels in BC cells with overexpressed SH3BP5-AS1. (F) qPCR analysis was done to testify IGF2BP2 knockdown efficiency. (G) qPCR and WB analyses were applied to measure VDAC2 expression in IGF2BP2-depleted cells. (H) In RIP assay, the level of VDAC2 precipitated in Anti-IGF2BP2 was detected in T24 and J82 cells transfected with pcDNA3.1 or pcDNA3.1-SH3BP5-AS1. (I) VDAC2 mRNA and protein levels were examined via qPCR and WB in the cells with transfection of sh-IGF2BP2 or sh-IGF2BP2 + pcDNA3.1-SH3BP5-AS1. **p < 0.01, n.s.: no significance.
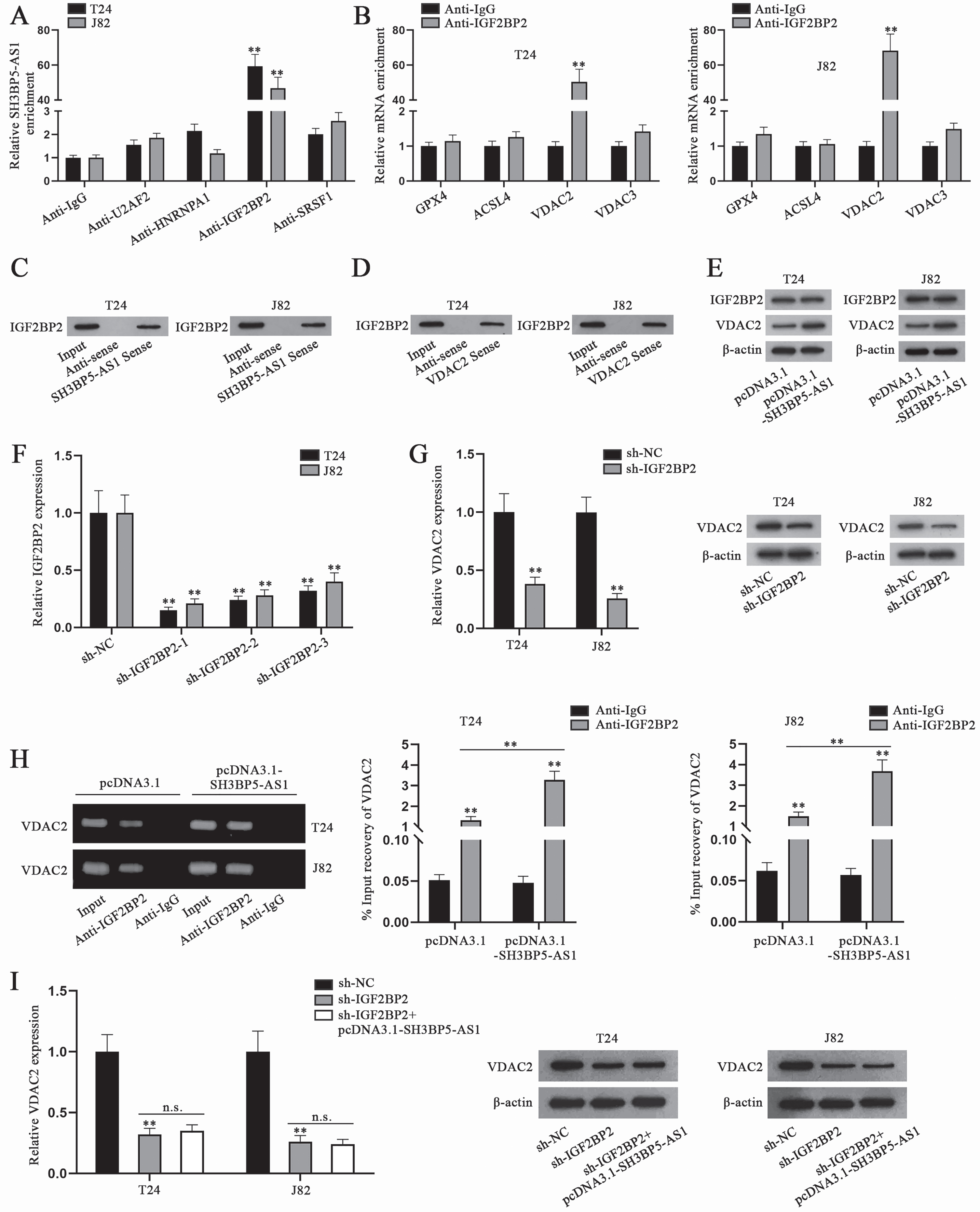
IGF2BP2 promotes the stability of VDAC2 in an m6A-dependent manner
Considering that IGF2BPs, including IGF2BP1/2/3, have been testified as m6A readers to enhance the stability of target mRNAs [23], we hypothesized that IGF2BP2 might also mediate the stability of VDAC2 through m6A modification. As depicted in Fig. 4A, VDAC2 enrichment in Anti-m6A was abundant, indicating the possibility of VDAC2 m6A modification. Then, the existence of m6A sites in VDAC2 was found after searching on SRAMP (https://www.cuilab.cn/sramp), and the two sites (site 1199 and site 1206) with highest prediction scores were further investigated in this study (Fig. 4B). Subsequently, IGF2BP2 overexpression efficiency was testified (Fig. 4C). To determine the specific methylation site of VDAC2 influenced by IGF2BP2, luciferase reporter assay was implemented. Based on the experimental results, the luciferase activity of pGL3-VDAC2 (m6A-1206 Mut) was hardly affected by IGF2BP2 up-regulation while that of pGL3-VDAC2 (m6A-Wt) and pGL3-VDAC2 (m6A-1199 Mut) was evidently strengthened under the same condition, reflecting that IGF2BP2 influenced m6A methylation of VDAC2 at site 1206 (Fig. 4D). RNA pulldown assay was carried out for further validation. As depicted in Fig. 4E, IGF2BP2 could be pulled down by biotinylated VDAC2 sense, but not by biotinylated VDAC2 antisense or biotinylated VDAC2 sense with mutated site 1206, which further confirmed the interaction between IGF2BP2 and VDAC21206. Moreover, in accordance to qPCR analysis, VDAC2 transcription in BC cells with IGF2BP2 depletion declined more quickly compared with the sh-NC group after 50mM β-amanitin treatment (Fig. 4F). To conclude, IGF2BP2 enhances the stability of VDAC2 mRNA via modulating its m6A methylation at site 1206 in BC cells.
Fig. 4
IGF2BP2 enhances VDAC2 mRNA stability in an m6A-dependent manner. (A) In RIP assay, VDAC2 enrichment in Anti-m6A was quantified via qPCR. (B) Bioinformatics analysis was done to predict the m6A methylation site on VDAC2. (C) IGF2BP2 overexpression efficiency was determined via qPCR analysis. (D) In luciferase reporter assay, cells were co-transfected with different luciferase reporter constructs and pcDNA3.1 or pcDNA3.1-IGF2BP2 to determine the methylation site on VDAC2. (E) After RNA pulldown assay, IGF2BP2 level pulled down by the indicated probes was measured via WB. (F) VDAC2 mRNA stability was evaluated at different time points in sh-NC- or sh-IGF2BP2-transfected cells after the addition of 50mM β-amanitin. **P < 0.01.
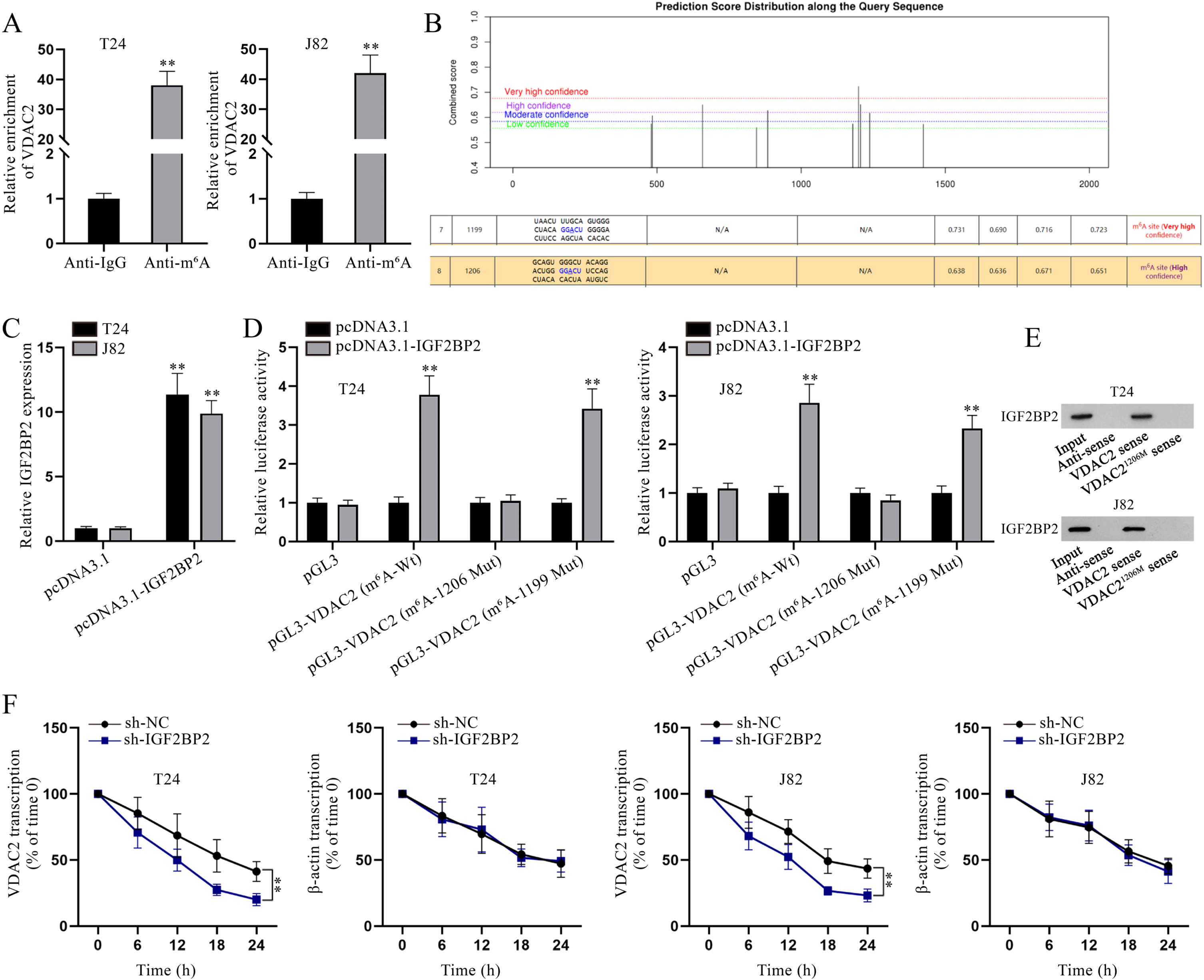
VDAC2 overexpression inhibits BC cell proliferation and accelerates BC cell apoptosis
To elucidate the role of VDAC2, we first assessed VDAC2 expression in BC cells and SV-HUC-1 cells. It was ascertained via qPCR that VDAC2 expression was aberrantly lower in BC cells compared with SV-HUC-1 cells (Fig. 5A). Then, VDAC2 was overexpressed in T24 and J82 cells (Fig. 5B). The impacts of VDAC2 overexpression on BC cell proliferation and apoptosis were then evaluated via CCK-8 and flow cytometry assays, respectively. In CCK-8 assay, cell viability was found to be mitigated as a result of pcDNA3.1-VDAC2 transfection (Fig. 5C). Flow cytometry assay unmasked BC cell apoptosis was exacerbated by VDAC2 augment (Fig. 5D). The above findings together reflect that VDAC2 is down-regulated in BC cells and VDAC2 overexpression can inhibit BC cell proliferation while promoting the cell apoptosis.
Fig. 5
VDAC2 is down-regulated in BC cells and VDAC2 overexpression impedes BC cell proliferation but promotes BC cell apoptosis. (A) VDAC2 expression in SV-HUC-1 and BC cell lines was detected via qPCR. (B) VDAC2 expression was measured in pcDNA3.1-VDAC2-transfected cells via qPCR. (C) CCK-8 was done to evaluate the proliferation of BC cells with VDAC2 overexpression. (D) The apoptotic ability of VDAC2-overexpressed cells was detected via flow cytometry assay. **p < 0.01.
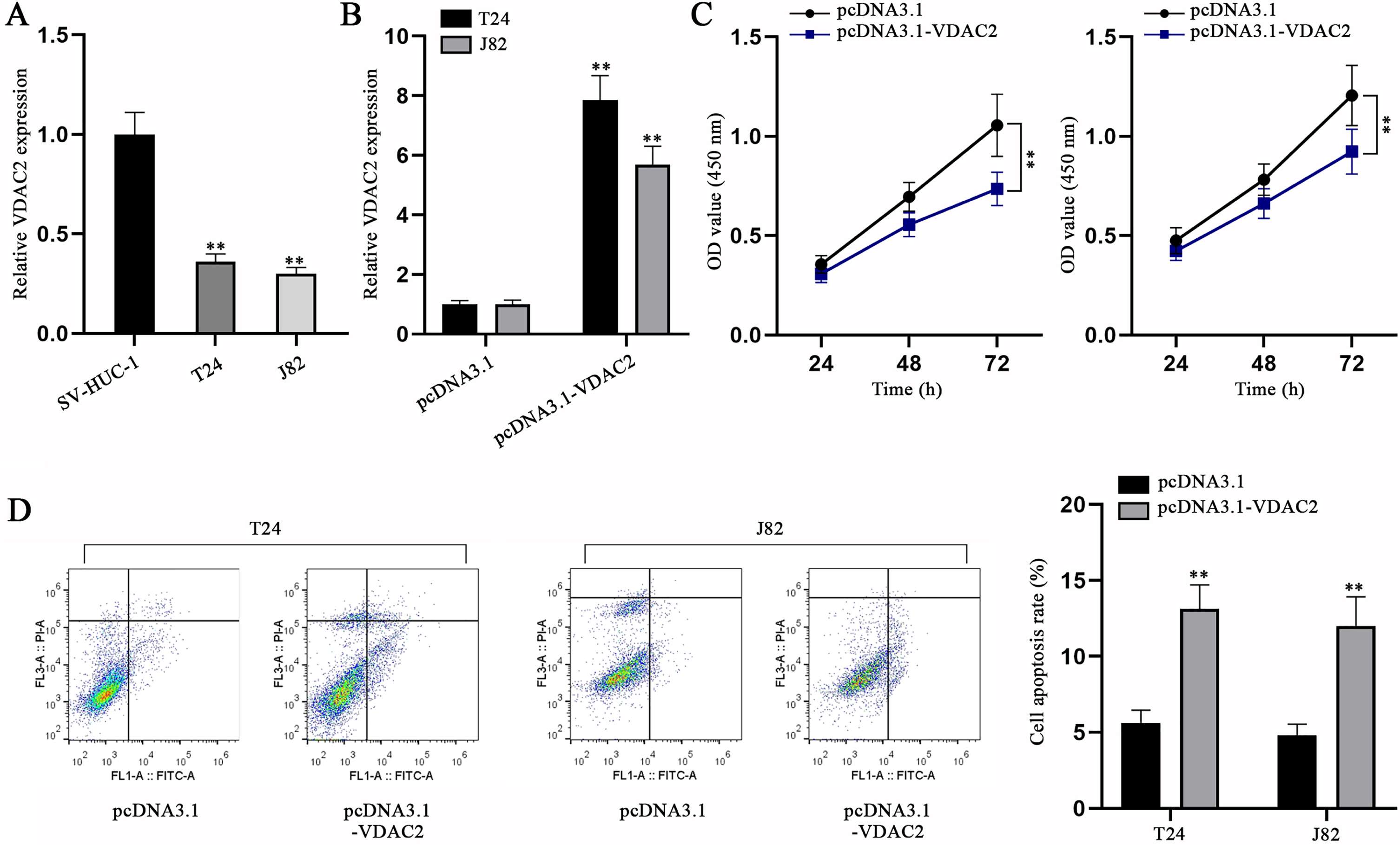
SH3BP5-AS1 modulates BC cell ferroptosis via VDAC2
To elucidate the validity of SH3BP5-AS1/VDAC2 axis in modulating BC cell ferroptosis, we designed and implemented rescue assays. VDAC2 knockdown efficiency was tested through qPCR in advance (Fig. 6A). In subsequent assays, sh-VDAC2-1 (termed as sh-VDAC2) with highest knockdown efficiency was applied. Then, we conducted rescue assays to probe into the impacts of SH3BP5-AS1/VDAC2 on cell ferroptosis. By analyzing the results, we found that the elevation in Fe2 + content induced by SH3BP5-AS1 overexpression could be recovered by co-transfection of sh-VDAC2 (Fig. 6B). Lipid ROS positive cell rate enhanced by SH3BP5-AS1 up-regulation was detected to be reversed by VDAC2 knockdown (Fig. 6C). To sum up, SH3BP5-AS1 can mediate BC cell ferroptosis via modulation on VDAC2.
Fig. 6
SH3BP5-AS1 exerts its promoting impacts on BC cell ferroptosis via VDAC2. (A) qPCR was applied to examine VDAC2 expression in the cells with transfection of sh-VDAC2-1/2/3. (B) Ferrous iron content in BC cells was detected with a microplate reader after the transfection of indicated plasmids including pcDNA3.1, pcDNA3.1-SH3BP5-AS1, and pcDNA3.1-SH3BP5-AS1 + sh-VDAC2. (C) Lipid ROS was measured in the cells with transfection of indicated plasmids. **p < 0.01.
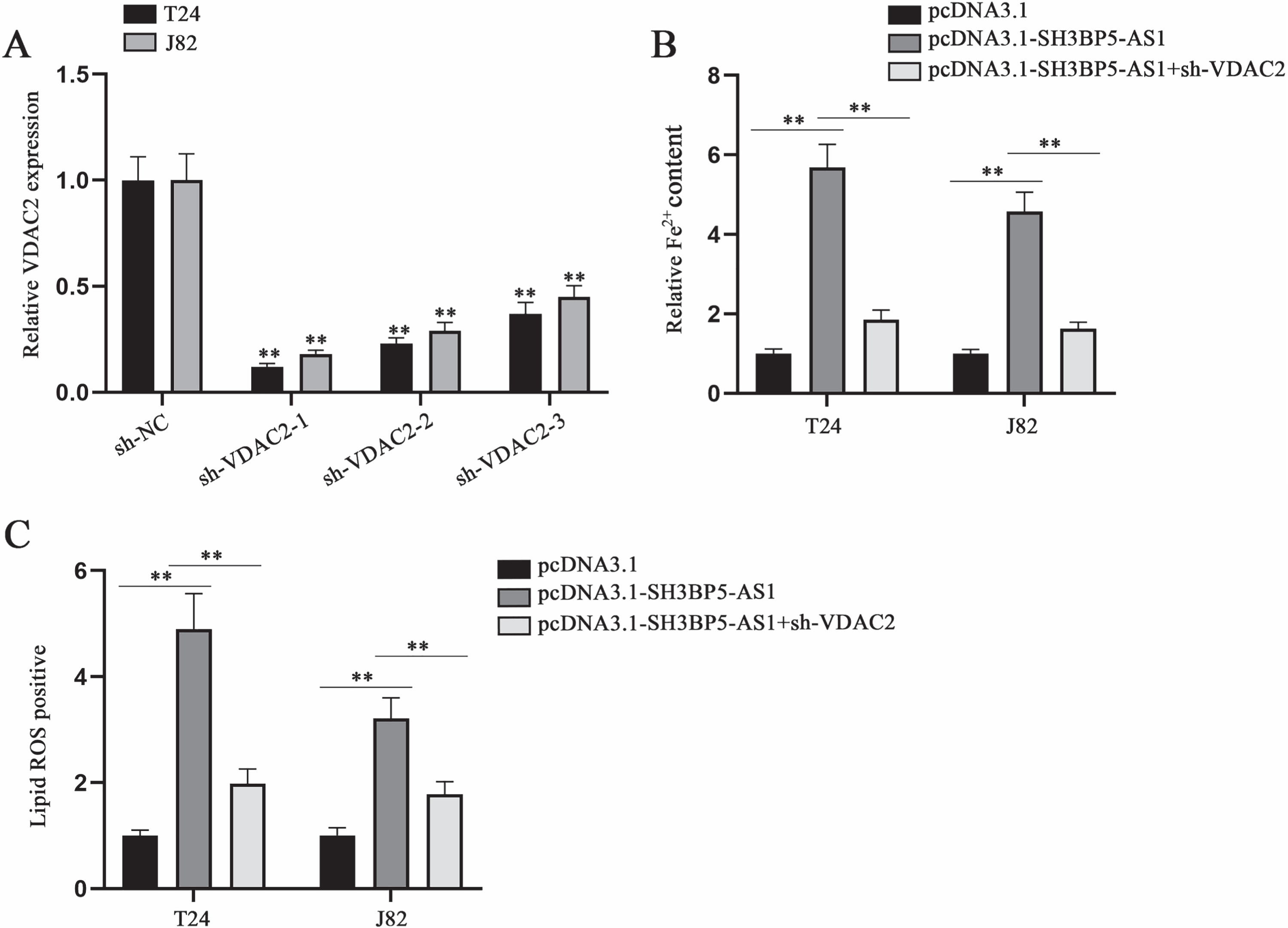
4DISCUSSION
In our study, the aberrantly low expression of SH3BP5-AS1 in BC cells was detected. Through functional assays, we confirmed overexpression of SH3BP5-AS1 could inhibit BC cell proliferation while promoting BC cell apoptosis. Existing studies have unveiled that lncRNAs could interact with RBPs to modulate the expression of downstream genes, thus influencing cellular processes [24]. Therefore, we assumed that SH3BP5-AS1 might also regulate downstream gene expression via the interaction with certain RBP to exert influence on BC cells. Considering the association between candidate RBPs potentially binding to SH3BP5-AS1 and key genes of ferroptosis, we also detected Fe2 + content and lipid ROS in BC cells with SH3BP5-AS1 overexpression and confirmed SH3BP5-AS1 overexpression could result in promoted ferroptosis. Through mechanism assays, accompanied with qPCR and WB, IGF2BP2 was confirmed to be the RBP recruited by SH3BP5-AS1 in BC cells to modulate the expression of VDAC2, the key ferroptosis gene.
Thereafter, we continued to probe into the regulatory mechanism involving IGF2BP2 and VDAC2. IGF2BP family has been repeatedly validated to maintain mRNA stability as m6A readers [25, 26]. To examine whether IGF2BP2 also influenced VDAC2 via m6A modulation, we first applied RIP assay to ensure the binding between IGF2BP2 and VDAC2 m6A site. Luciferase reporter assay, RNA pulldown assay and mRNA stability assay further validated that IGF2BP2 could methylate VDAC2 at site 1206 to stabilize VDAC2 mRNA.
The role of VDAC2 was also deeply examined. We found that VDAC2 expression in BC cells was also aberrantly low. VDAC2 has been frequently reported as a crucial effector of ferroptosis [27]. However, there are also studies unveiling that VDAC2 could affect tumor cell apoptosis [28]. Considering that SH3BP5-AS1 could affect BC cell proliferation and apoptosis, we also conducted functional experiments and validated that cell proliferation was hampered by VDAC2 overexpression while cell apoptosis was largely increased. Finally, the validity of SH3BP5-AS1/VDAC2 in influencing BC cell ferroptosis was ascertained through rescue experiments, as VDAC2 knockdown rescued the exacerbated ferroptosis of BC cells induced by SH3BP5-AS1 up-regulation.
Until now, we could draw the conclusion that SH3BP5-AS1 recruited IGF2BP2 to enhance VDAC2 mRNA stability, thus promoting the ferroptosis of BC cells. This study successfully revealed the tumor suppressive role of SH3BP5-AS1 in BC, and verified the correlation between SH3BP5-AS1 and BC cell ferroptosis. Although these findings might be further confirmed with involvement of clinical samples and animal experiments, a more comprehensive understanding of molecular mechanisms in BC could still be achieved, which might ultimately contribute to the development of novel biomarkers for BC diagnosis or treatment.
ACKNOWLEDGMENTS
We sincerely thank for the help provided by all lab personnel in this research.
FUNDING
The authors report no funding.
AUTHOR CONTRIBUTIONS
Yong Shao devoted to research design and wrote the paper.
Yong Shao, Yunhui Chan and Rong Zhao were responsible for experiment and figures.
All authors analyzed the data, read and approved the final manuscript.
CONFLICT OF INTEREST
Yong Shao has no conflict of interest to report.
Yunhui Chan has no conflict of interest to report.
Rong Zhao has no conflict of interest to report.
SUPPLEMENTARY MATERIAL
[1] The supplementary material is available in the electronic version of this article: https://dx.doi.org/10.3233/BLC-211629.
REFERENCES
[1] | Quan J , Pan X , Zhao L , Li Z , Dai K , Yan F , et al., LncRNA as a diagnostic and prognostic biomarker in bladder cancer: a systematic review and meta-analysis. OncoTargets and Therapy (2018) ;11: :6415–24. |
[2] | Martinez Rodriguez RH , Buisan Rueda O , Ibarz L , Bladder cancer: Present and future. Medicina Clinica (2017) ;149: (10):449–55. |
[3] | Babjuk M , Burger M , Compérat EM , Gontero P , Mostafid AH , PalouJ , et al., European Association of Urology Guidelines onNon-muscle-invasive Bladder Cancer (TaT1 and Carcinoma In Situ) -Update. European Urology (2019) ;76: (5):639–57. |
[4] | Clark PE , Spiess PE , Agarwal N , Bangs R , Boorjian SA , Buyyounouski MK , et al., NCCN Guidelines Insights: Bladder Cancer, Version 2. Journal of the National Comprehensive Cancer Network: JNCCN (2016) ;14: (10):1213–24. |
[5] | Zhang C , Wang W , Lin J , Xiao J , Tian Y , lncRNA CCAT1 promotes bladder cancer cell proliferation, migration and invasion. International braz j urol: official journal of the Brazilian Society of Urology (2019) ;45: (3):549–59. |
[6] | Cao HL , Liu ZJ , Huang PL , Yue YL , Xi JN , lncRNA-RMRP promotes proliferation, migration and invasion of bladder cancer via miR-206. European Review for Medical and Pharmacological Sciences (2019) ;23: (3):1012–21. |
[7] | Luo H , Xu C , Le W , Ge B , Wang T , lncRNA CASC11 promotes cancer cell proliferation in bladder cancer through miRNA-150. Journal of Cellular Biochemistry (2019) ;120: (8):13487–93. |
[8] | Zheng R , Du M , Wang X , Xu W , Liang J , Wang W , et al., Exosome-transmitted long non-coding RNA PTENP1 suppresses bladder cancer progression. Molecular Cancer (2018) ;17: (1):143. |
[9] | Utnes P , Løkke C , Flægstad T , Einvik C , Clinically RelevantBiomarker Discovery in High-Risk Recurrent Neuroblastoma. CancerInformatics (2019) ;18: :1176935119832910. |
[10] | Cao R , Yuan L , Ma B , Wang G , Tian Y , Immune-related long non-coding RNA signature identified prognosis and immunotherapeutic efficiency in bladder cancer (BLCA). Cancer Cell International (2020) ;20: :276. |
[11] | Han C , Liu Y , Dai R , Ismail N , Su W , Li B , Ferroptosis and Its Potential Role in Human Diseases. Frontiers in Pharmacology (2020) ;11: , 239. |
[12] | Xie Y , Hou W , Song X , Yu Y , Huang J , Sun X , et al Ferroptosis: process and function. Cell Death and Differentiation (2016) ;23: (3):369–79. |
[13] | Lu B , Chen XB , Ying MD , He QJ , Cao J , Yang B , The Role of Ferroptosis in Cancer Development and Treatment Response. Frontiers in Pharmacology (2017) ;8: , 992. |
[14] | Li Y , Feng D , Wang Z , Zhao Y , Sun R , Tian D , et al., Ischemia-induced ACSL4 activation contributes to ferroptosis-mediated tissue injury in intestinal ischemia/reperfusion. Cell Death and Differentiation (2019) ;26: (11):2284–99. |
[15] | Stockwell BR , Jiang X , A Physiological Function for Ferroptosis in Tumor Suppression by the Immune System. Cell Metabolism (2019) ;30: (1):14–5. |
[16] | Mao C , Wang X , Liu Y , Wang M , Yan B , Jiang Y , et al., A G3BP1-Interacting lncRNA Promotes Ferroptosis and Apoptosis in Cancer via Nuclear Sequestration of p53. Cancer Research (2018) ;78: (13):3484–96. |
[17] | Yang Y , Tai W , Lu N , Li T , Liu Y , Wu W , et al., lncRNA ZFAS1 promotes lung fibroblast-to-myofibroblast transition and ferroptosis via functioning as a ceRNA through miR-150-5p/SLC38A1 axis. Aging (2020) ;12: (10):9085–102. |
[18] | Zhang H , Deng T , Liu R , Ning T , Yang H , Liu D , et al., CAF secreted miR-522 suppresses ferroptosis and promotes acquired chemo-resistance in gastric cancer. Molecular Cancer (2020) ;19: (1):43. |
[19] | Zhang M , Sun Y , Huang CP , Luo J , Zhang L , Meng J , et al., Targeting the Lnc-OPHN1-5/androgen receptor/hnRNPA1 complex increases Enzalutamide sensitivity to better suppress prostate cancer progression. Cell Death & Disease (2021) ;12: (10):855. |
[20] | Qi W , Li Z , Xia L , Dai J , Zhang Q , Wu C , et al., LncRNA GABPB1-AS1 and GABPB1 regulate oxidative stress during erastin-induced ferroptosis in HepG2 hepatocellular carcinoma cells. Scientific Reports (2019) ;9: (1):16185. |
[21] | Liu Z , Wang Q , Wang X , Xu Z , Wei X , Li J , Circular RNA cIARS regulates ferroptosis in HCC cells through interacting with RNA binding protein ALKBH5. Cell Death Discovery (2020) ;6: , 72. |
[22] | Li J , Cao F , Yin HL , Huang ZJ , Lin ZT , Mao N , et al., Ferroptosis: past, present and future. Cell Death & Disease (2020) ;11: (2):88. |
[23] | Huang H , Weng H , Sun W , Qin X , Shi H , Wu H , et al., Recognition of RNA N(6)-methyladenosine by IGF2BP proteins enhances mRNA stability and translation. Nature Cell Biology (2018) ;20: (3):285–95. |
[24] | Xu Y , Wu W , Han Q , Wang Y , Li C , Zhang P , et al., New Insights into the Interplay between Non-Coding RNAs and RNA-Binding Protein HnRNPK in Regulating Cellular Functions. Cells (2019) ;8: (1). |
[25] | Gu Y , Niu S , Wang Y , Duan L , Pan Y , Tong Z , et al., DMDRMR-Mediated Regulation of m(6)A-Modified CDK4 by m(6)A Reader IGF2BP3 Drives ccRCC Progression. Cancer Research (2021) ;81: (4):923–34. |
[26] | Yang Z , Wang T , Wu D , Min Z , Tan J , Yu B , RNA N6-methyladenosine reader IGF2BP3 regulates cell cycle and angiogenesis in colon cancer. Journal of Experimental & Clinical Cancer Research: CR (2020) ;39: (1):203. |
[27] | Yang Y , Luo M , Zhang K , Zhang J , Gao T , Connell DO , et al., Nedd4 ubiquitylates VDAC2/3 to suppress erastin-induced ferroptosis in melanoma. Nature Communications (2020) ;11: (1):433. |
[28] | Chin HS , Li MX , Tan IKL , Ninnis RL , Reljic B , Scicluna K , et al., VDAC2 enables BAX to mediate apoptosis and limit tumor development. Nature Communications (2018) ;9: (1):4976. |