The evolution of biomedical vibrational spectroscopy: A personal perspective
1.Preamble
When my colleague Parvez Haris asked me to write a historical review on the evolution of biomedical vibrational spectroscopy I consented, but now I need to clarify what this review is about and what it is not. It is my personal belief that biomedical vibrational spectroscopy has yet to reach its full potential and therefore I will not restrict myself to recounting its past history but will look ahead to its future and where it may still be evolving. Accordingly, my review will comprise two parts. As yours truly has now joined the league of octogenarians, in the first part I plan to share with the readers my personal recollections of the very early days of vibrational bio-spectroscopy which I was privileged to witness and be part of. In the second part I intend to peer into the crystal ball and speculate on new applications in the medical sciences, envisioning vibrational spectroscopy as a tool for the exploration of the human mind to probe psychosomatic diseases and emotional disorders.
2.The arduous road to biomedical vibrational spectroscopy: Where did we come from?
As is often the case with historical reviews it can be difficult to precisely pinpoint a beginning since this depends on how far back the reviewer is prepared to go. The exploitation of vibrational spectroscopy for use in medicine clearly relies on earlier applications in biology, which go back to previous uses in chemistry, which in turn rest on the solid foundations of vibrational spectroscopy in physics. Having said this I now wish to refer the reader to a few reviews which deal with this progression of vibrational spectroscopy from physics to chemistry to biology to medicine. Four pre-eminent publications, written in 1985 [14], 2009 [1], 2012 [11] and 2014 [4] supply over 700 bibliographic references (with some duplications) that comprehensively document this evolution, allowing this reviewer to focus on events he has personally witnessed and experienced over the past 50+ years.
It is fair to state that until the 1950s vibrational spectroscopy was the purview of physics, when a growing number of chemists began to employ it regularly for the elucidation of molecular structures. Around the same time the chemical industry embraced vibrational spectroscopy as an important analytical tool, moving it out of the arena of academic research. The progression of vibrational spectroscopy from physics to chemistry was complete by 1971 when Gerhard Herzberg, much to his own surprise, was awarded the Nobel Prize for Molecular Spectroscopy in Chemistry and not in Physics. The citation of the Nobel Committee from 1971 sums it up:
“This year’s Nobel prize winner in Chemistry, Dr Gerhard Herzberg, is considered to be the world’s foremost molecular spectroscopist, his institute in Ottawa is the undisputed centre for such research. One may now ask why Herzberg – originally a physicist and most famous amongst astrophysicists – was awarded the Nobel Prize in Chemistry. The explanation is that in the 1950s molecular spectroscopy had progressed so far that one could begin to study even complicated systems of great chemical interest”.
The next step, from chemistry to biology, followed swiftly and happened in the late 1970s and early 1980s when more and more vibrational spectroscopists discovered the aqueous world of biomolecules. Incidentally, in 1959 when this author wrote his PhD thesis on infrared spectroscopic studies of natural products, the term biomolecule had not yet been coined. The fact that these biomolecules or molecules of life were selected through evolution for their fitness to perform specific biological functions most certainly helped fuel the interest of many scientists at the time. It is perhaps also a reason why the spectroscopy of biomolecules remains a challenging topic to this day. Throughout the 1980s biological infrared and Raman spectroscopy rapidly gained ground with numerous research groups emerging in different parts of the world.
It will not come as a great surprise that after the successful use of vibrational spectroscopy in the biological sciences many aficionados (both young and seasoned) then turned their attention to applications in medicine. Two early reports from 1949 [3] and 1952 [34] are often cited as the first studies of human and animal tissues, however in view of the rudimentary instrumental capabilities of that time they are of little scientific value today. Biomedical applications of vibrational spectroscopy only began in earnest in the late-1980s, taking off in the 1990s and expanding rapidly in the New Millennium. Some applications, particularly those dealing with the diagnosis or prognosis of disease, became realistic only in the 21st century after the dawn of a new discipline, bio-informatics.
Biomedical vibrational spectroscopy rests on two pillars: infrared and Raman spectroscopy. It is well known that throughout their history these two techniques constantly competed with each other. Initially infrared spectroscopy had the field for itself but then the introduction of laser sources for Raman spectroscopy in the mid-1960s tilted the balance in favour of the latter, particularly for studies which used the evolving Resonance Raman technique. The advent of interferometric FT-IR spectrometers in the mid-1970s then swayed the pendulum back in favour of infrared spectroscopy. It is gratifying to see that today both Raman and infrared techniques are used equally, often by the same groups or labs.
When this author arrived at the National Research Council (the NRC) in Ottawa in the late 1960s there were three dedicated spectroscopy laboratories operating at the NRC. These research labs were located at 100 Sussex Drive in Ottawa, an address well known to the spectroscopic community all over the world, an edifice to which the locals referred to as the Temple of Science (shown here in Fig. 1).
Fig. 1.
The Temple of Science in Ottawa, built in the 1930s (from an old NRC pamphlet).
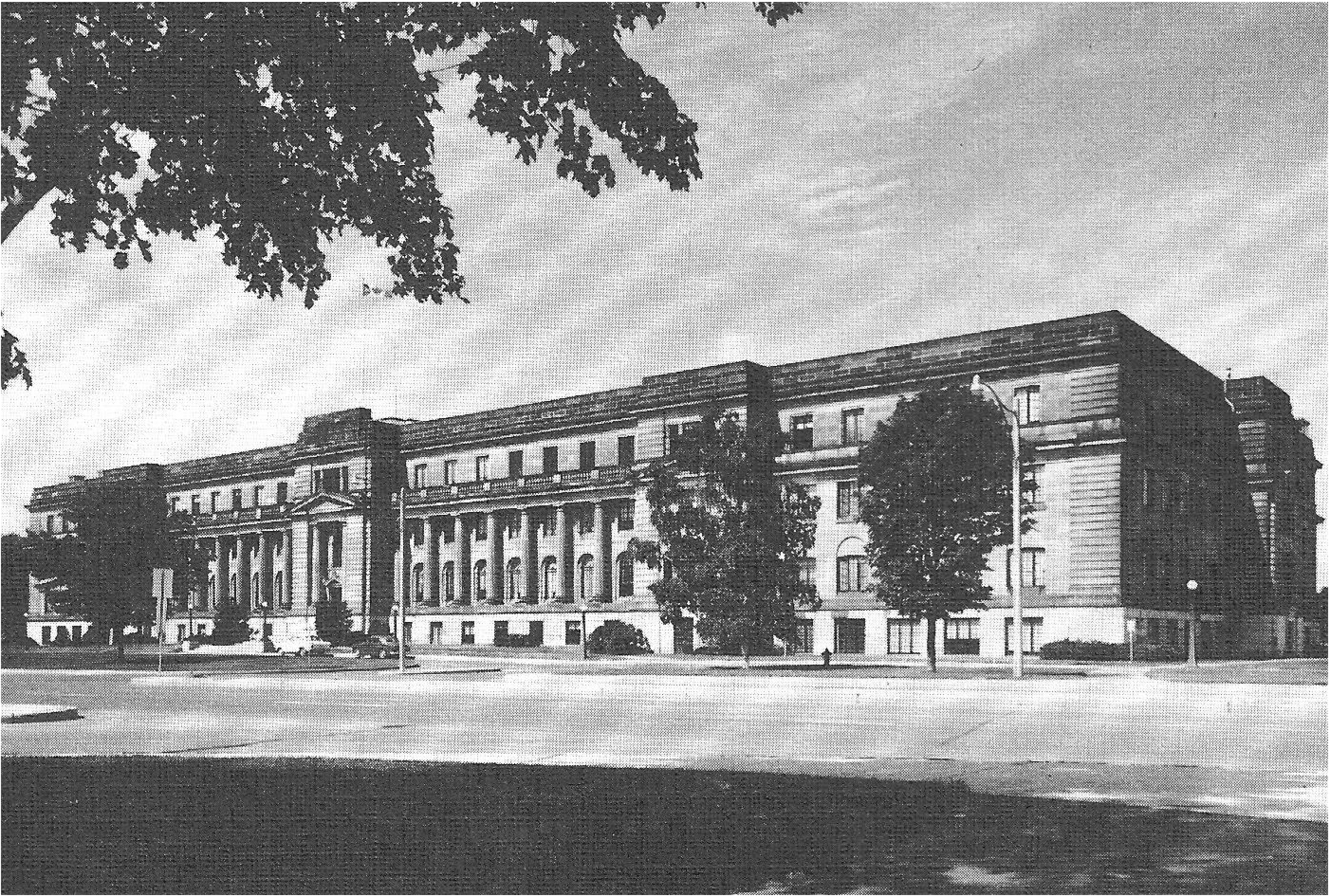
The best known of the three spectroscopy orchestras performing in this Temple of Science was that of Gerhard Herzberg in the Division of Physics. Herzberg had fled Nazi Germany in the mid-1930s and arrived at the NRC in Ottawa in 1948 via the University of Saskatoon and the University of Chicago. Meanwhile Harold Bernstein and Norman Jones, who came to the NRC in Ottawa immediately after WWII, had already established reputable research laboratories in the Division of Chemistry. With such a critical mass the NRC was in an excellent position to attract aspiring young postdocs interested in molecular spectroscopy, including this author. In his Nobel lecture, Harry Kroto, also an NRC postdoctoral fellow from the mid-1960s, referred to the NRC in Ottawa as a true Mecca of Spectroscopy, recognising its stimulating atmosphere as a contributing factor to his Prize. Many other Nobel Laureates and distinguished spectroscopists visited or worked in this Spectroscopy Temple in Ottawa at one time or another during their career. David Buckingham for instance, was a regular visitor from Cambridge and when we spotted his lanky figure pacing up and down the corridor, we knew that summer had arrived in Ottawa. An interesting visitor was Subramanyan Chandrasekar, the nephew of Raman. Chandra and his sociable wife Lalitha often came up from Chicago to visit the Herzbergs in Ottawa. Like his uncle Raman, Chandra was a gifted speaker and kept everybody spellbound when he talked about black holes. We were all pleased to learn that he was awarded the 1983 Nobel Prize in Physics. In 1994, John Polanyi, an early NRC postdoctoral fellow with Herzberg and recipient of the 1986 Nobel Prize in Chemistry for the invention of the infrared laser, convened a meeting of twelve Nobel Laureates in Canada to honour Herzberg’s 90’ birthday (for names and Prize details see Fig. 2). This get-together was a true celebration of minds and a coronation of Herzberg’s long life (1904–1999) which spanned most of the 20th Century. Many of the Nobel Laureates delighted the budding scientists in the audience by sharing interesting stories from their own life. Charlie Townes for instance told the audience that when he first wanted to patent the invention of the laser, he was told “this is such an esoteric invention, it will never, ever be of any practical importance”. Michael Smith on the other hand urged the young scientists not to be easily discouraged, revealing that his original manuscript describing the polymerase chain reaction (PCR) which eventually netted him the Prize, was turned down not once but twice so that he had to publish it in a third rate journal.
Fig. 2.
A celebration of Minds. Seated, from left to right: Gerhard Herzberg (Canada, Chemistry, 1971), George Porter (UK, Chemistry, 1967), James Watson (US, Medicine, 1962), Ilya Prigogine (Belgium, Chemistry, 1977). Standing, from left to right: Max Perutz (UK, Chemistry, 1962), Michael Smith (Canada, Chemistry, 1993), Dudley Herschbach (US, Chemistry, 1986), Bertram Brockhouse (Canada, Physics, 1994), John Polanyi (Canada, Chemistry, 1986), Christian de Duve (Belgium, Medicine, 1974), Charles Townes (US, Physics, 1964), Henry Kendall (US, Physics, 1990) (personal photograph). (Colors are visible in the online version of the article; http://dx.doi.org/10.3233/BSI-150118.)
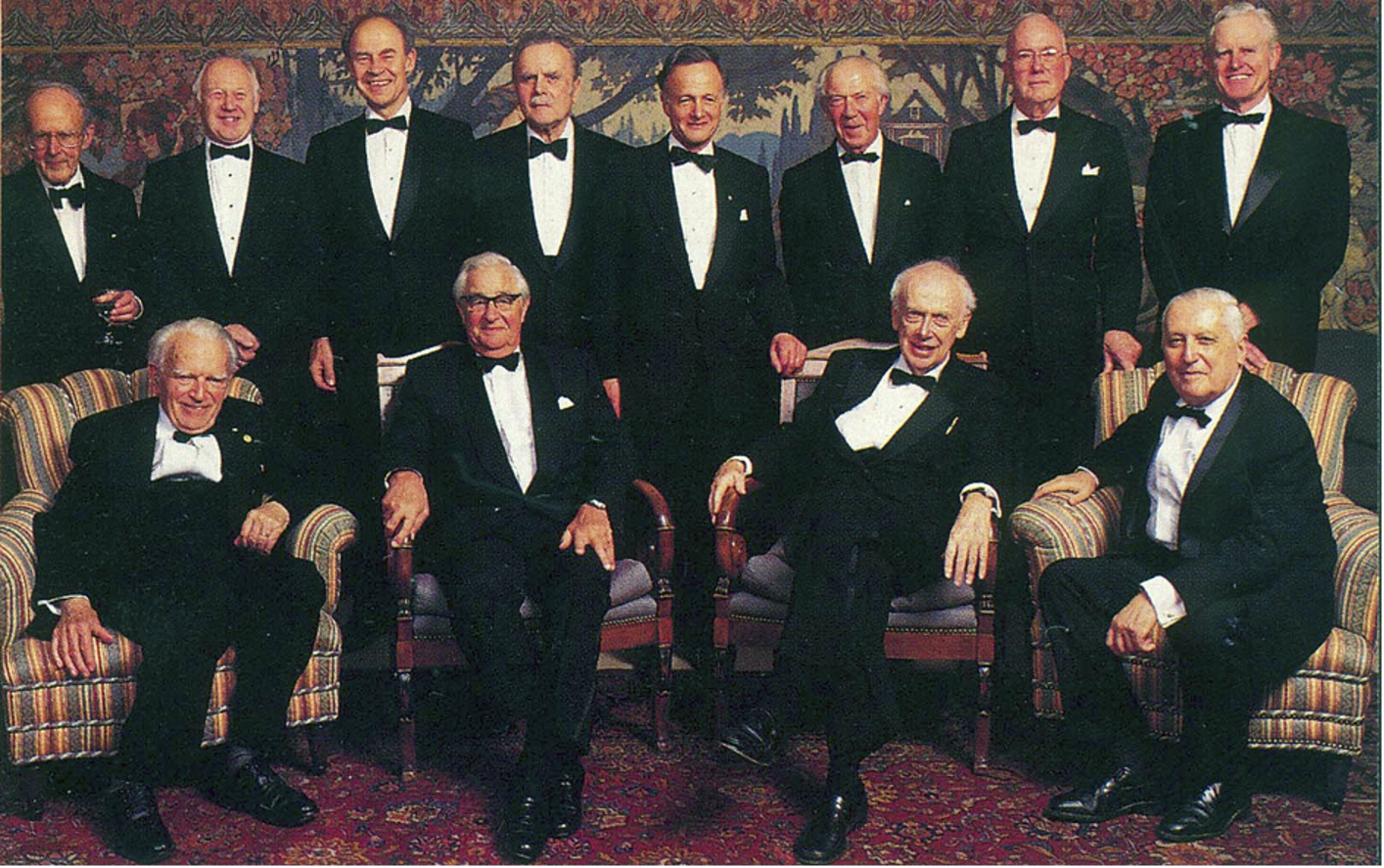
As a trained chemist, albeit a physical chemist, this author commenced his NRC postdoctoral fellowship in the Division of Chemistry with Norman Jones in 1968. I first met Jones, Bernstein and Herzberg in 1963 at the European Molecular Spectroscopy Congress in Budapest and was so impressed by their personalities that only a few years later I found myself at the NRC in Ottawa. The group under the direction of Dr Richard Norman Jones was engaged in the study of infrared and ultraviolet spectra of large molecules with particular interest in molecules of biological significance. Jones had shown an early interest in biomolecules, his PhD thesis from 1939 in Manchester dealt with the structure of vitamin D and as consultant to the Sloan Kettering Institute for Cancer Research in New York he initiated a steroid hormone metabolism program based on infrared spectra. However, Jones’ true legacy remains that of having married infrared spectroscopy to the computer [15]. Between 1968 and 1986 his spectroscopy lab in Ottawa produced some 50 computer programs for infrared spectroscopy which were disseminated to the spectroscopic community at large for a token fee that barely covered the cost of the magnetic tape on which they were distributed. Many instrument manufacturers of the time freely incorporated these into their commercial data reduction programs. Being an effective communicator and an influential voice in international science, Jones helped establish the IUPAC Commission on Molecular Structure and Spectroscopy and the Committee on Data for Science and Technology (CODATA). Norman however was not the retiring type of scientist and became a regular visitor in his former lab at NRC, as can be seen from Fig. 3.
Fig. 3.
Norman Jones drops in on his former lab. Left to right: D. Moffatt (technician), H. Casal (postdoc), N. Jones (retired emeritus), S. Capes (student), H. Mantsch (author) and S. Surewicz (research associate). Note the NOVA data station in the foreground and the rack of magnetic tapes and disks in the background (personal photograph). (Colors are visible in the online version of the article; http://dx.doi.org/10.3233/BSI-150118.)
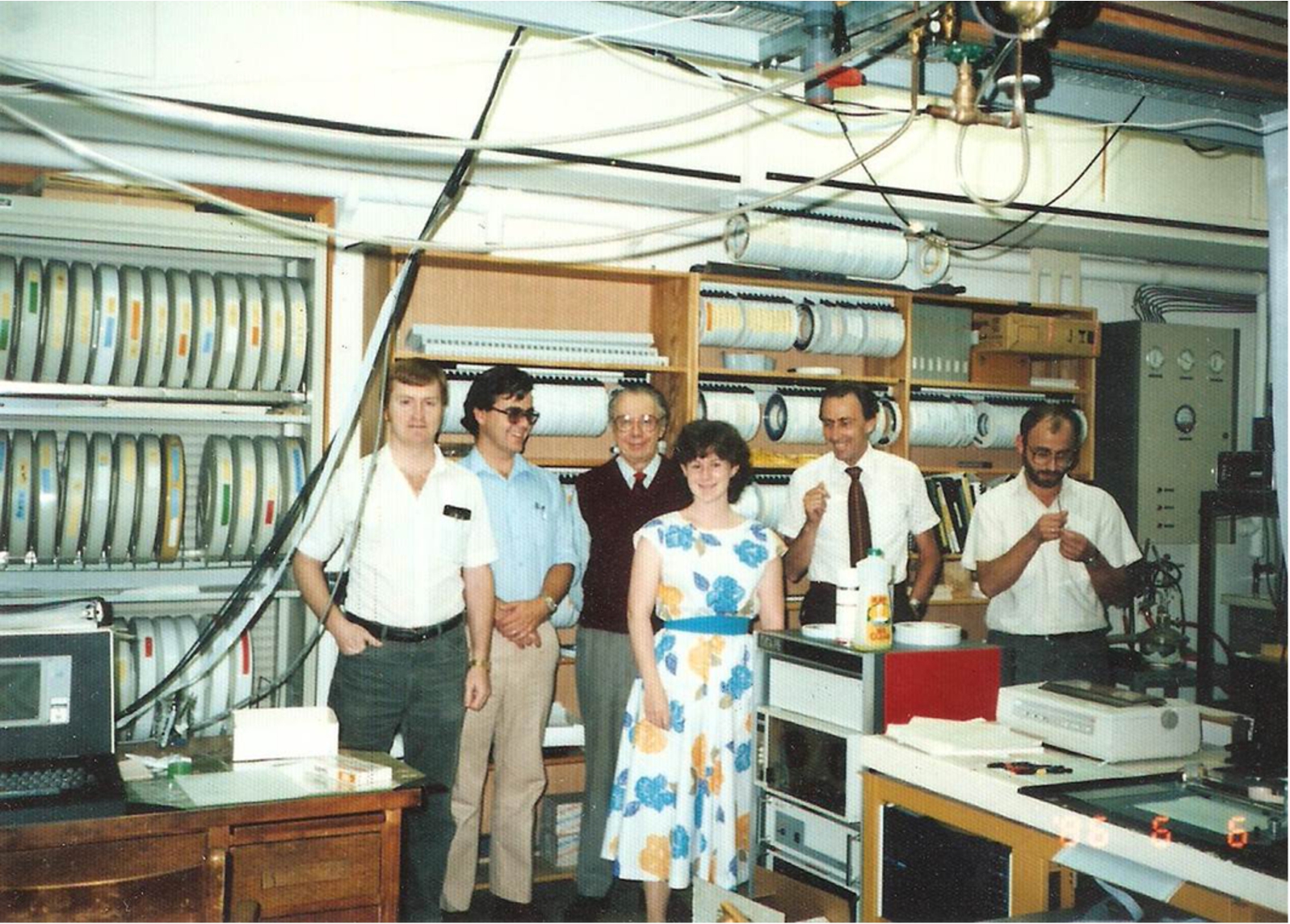
The second spectroscopy group in the Division of Chemistry was led by Dr Harold Bernstein and focussed on Raman spectra of simple molecules with emphasis on Raman band intensities which at that time were only poorly understood. Bernstein is perhaps best known to the international vibrational spectroscopic community for having launched the First International Conference on Raman Spectroscopy (ICORS) in 1969 in Ottawa. He was also the co-founder and co-editor of the Journal of Raman Spectroscopy. Though Bernstein found biomolecules a bit too large for his taste he was nonetheless receptive to the interest shown by his coworkers and postdocs and encouraged them to get involved in the study of complex biomolecules. In the mid-1960s his lab pioneered the emerging field of Resonance Raman spectroscopy for which Ottawa was soon to become an international hub. Paul Carey, who wrote a first review on Resonance Raman spectroscopy [7], Wolfgang Kiefer and Rich Mendelsohn are only three representative names standing for the many spectroscopists who spent time in Bernstein’s lab and who later established reputations of their own, so that the work started in Ottawa was continued in many places around the world.
The two spectroscopy groups in the Division of Chemistry were equipped with the latest instruments of low and medium resolution which were augmented by the high and ultra-high resolution instruments located in Herzberg’s Division of Physics. The research interests of the three spectroscopy groups were focused on different problems, however since we were all located in the same building there was much cross-fertilization, the scientists readily co-operated by lending equipment, helping with techniques and even joining forces on research topics of common interest. A fortuitous consequence of this co-location was sharing the same cafeteria where the three spectroscopy groups always took a break for afternoon tea at 3 o’clock to discuss science and other topics. The groups usually sat apart from each other but readily mixed when lively discussions broke out at one of the tables. Visitors were always invited to tea and often became a focal point.
Following Norman Jones’ and Harold Bernstein’s retirement from NRC in 1978 this author was privileged to be charged with heading both research groups. My first step after merging the two groups was to focus the research activities entirely on applications of vibrational spectroscopy in the biosciences. This raised a few eyebrows in the Division of Biological Sciences, however since the latter was heavily involved in exploiting the growing field of Magnetic Resonance Spectroscopy, rather than competing with each other, we collaborated and even published joint papers using multimodal spectroscopies [28]. My second radical move was to donate the brand-new Perkin Elmer 180 spectrometer to the University of Ottawa (much to the chagrin of Norman Jones who had purchased it less than a year ago). With my generous start-up funds I immediately proceeded to purchase an FTS-14 Digilab FT-IR spectrometer and not wanting to wait (I had seen what the FT-IR instruments could do in the hands of a Jim Alben or Jack Koenig) we pulled a few strings to take delivery of one of the next machines that came off the Digilab production line in Boston, allowing us to publish a first paper as early as 1978 [5].
It is fair to state that the advent of interferometric FT-IR spectroscopy had a greater impact in biology and in the life sciences than it had for chemistry and the physical sciences. The first category of biomolecules we turned our attention to were the lipids, or fats, as biochemists like to refer to them. There were reasons for this: First, it was an important research area in the Division of Biological Sciences, meaning that we could draw on their experience with this class of biomolecules, and also helping us apply vibrational spectroscopy to address the right questions. Secondly, the make-up of the lipid molecules with two chemically distinct constituents, a hydrophilic polar head group and two hydrophobic hydrocarbon chains, render them ideal for vibrational spectroscopic studies. Perhaps a third incentive for this choice came from my friend and competitor at the time, Dennis Chapman (see Fig. 4) who initiated the study of lipid polymorphism while at the industrial laboratories of Unilever in the UK, using conventional dispersive instruments (Perkin Elmer was next door to Unilever).
Fig. 4.
The author (on the left) in discussion with Dennis Chapman (on the right) over breakfast at the 1987 ECSBM Conference in Freiburg, Germany (personal photograph). (Colors are visible in the online version of the article; http://dx.doi.org/10.3233/BSI-150118.)
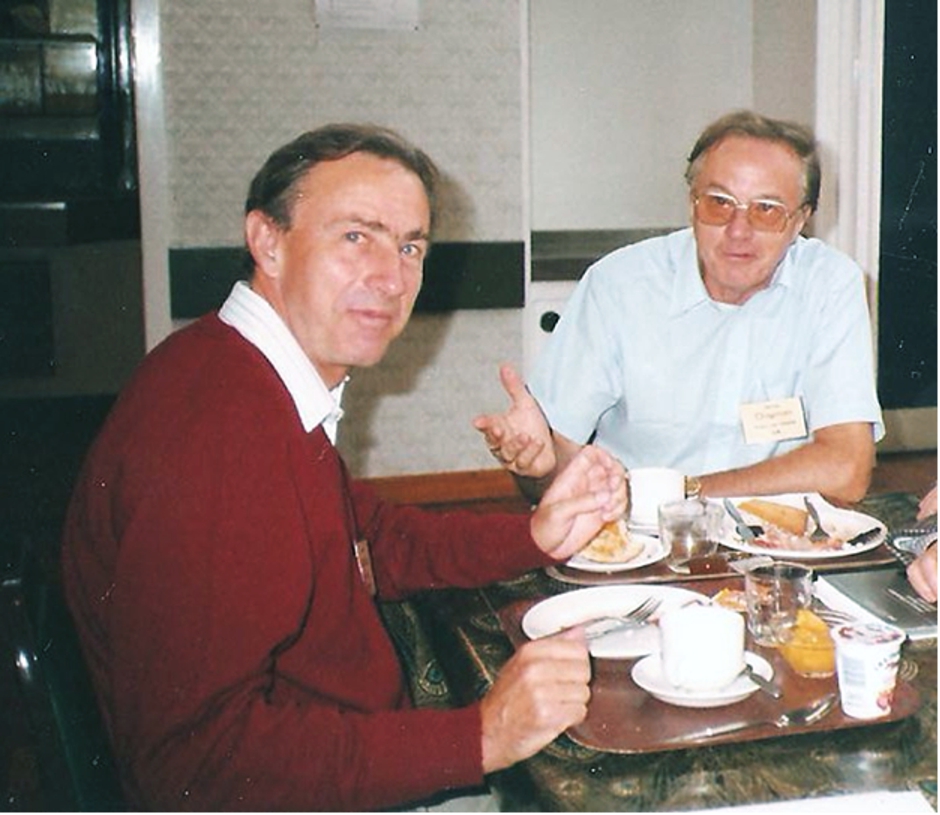
Being part of such a large research organization as NRC offered certain advantages. A particular research group dedicated solely to isotopic labelling welcomed challenging requests from us such as O-18 substitutions in the lipid ester groups or calling for lipids with per-deuterated sn-1 or sn-2 acyl chains [31]. Another group at NRC used high-pressure infrared and Raman spectroscopy to look into the various types of ice (an appropriate subject for Canada), so when Patrick Wong a member of that group transferred to our lab in 1980 he brought along a new tool for probing order-disorder transitions in biological systems. This allowed us to explore lipid polymorphism by inducing phase transitions through an increase in pressure as opposed to the conventional way of decreasing the temperature [35].
A dividend of interferometric FT-IR spectroscopy which was waiting to be cashed-in on was that many data processing operations now could be performed in Fourier space on the raw interferograms. Here we hit the jackpot in December 1979, when a young postdoc from Finland Jyrki Kauppinen joined our Ottawa group. When Jyrki, a high resolution spectroscopist by training, was confronted with the condensed phase infrared spectra of biological samples, he complained bitterly. I told him that if he did not like those broad featureless bands there was no point in complaining, but instead he had better find a way to deal with this – and so he did. For months on end Jyrki and one of our talented technical officers, Douglas Moffatt, “played” the computers with little to show for. Figure 5 puts a human face to those two individuals. Then, all of a sudden the break-through came, leading to three germane publications in rapid succession [16–18]. The most successful of these mathematical operations in Fourier space was a procedure we termed Fourier Self-Deconvolution (FSD), which provided spectral resolution enhancement by means of band-narrowing. A similar routine for band narrowing termed Fourier Derivation comprised the computation of derivatives in Fourier space which permitted the operator to adjust the degree of derivation to the signal-to-noise ratio in a particular spectrum. Since we did not patent these procedures they were quickly snatched up by various instrument manufacturers who incorporated them freely into their software packages.
Fig. 5.
Jyrki Kauppinen (on the left) and Douglas Moffatt (on the right) in action (personal photograph). (Colors are visible in the online version of the article; http://dx.doi.org/10.3233/BSI-150118.)
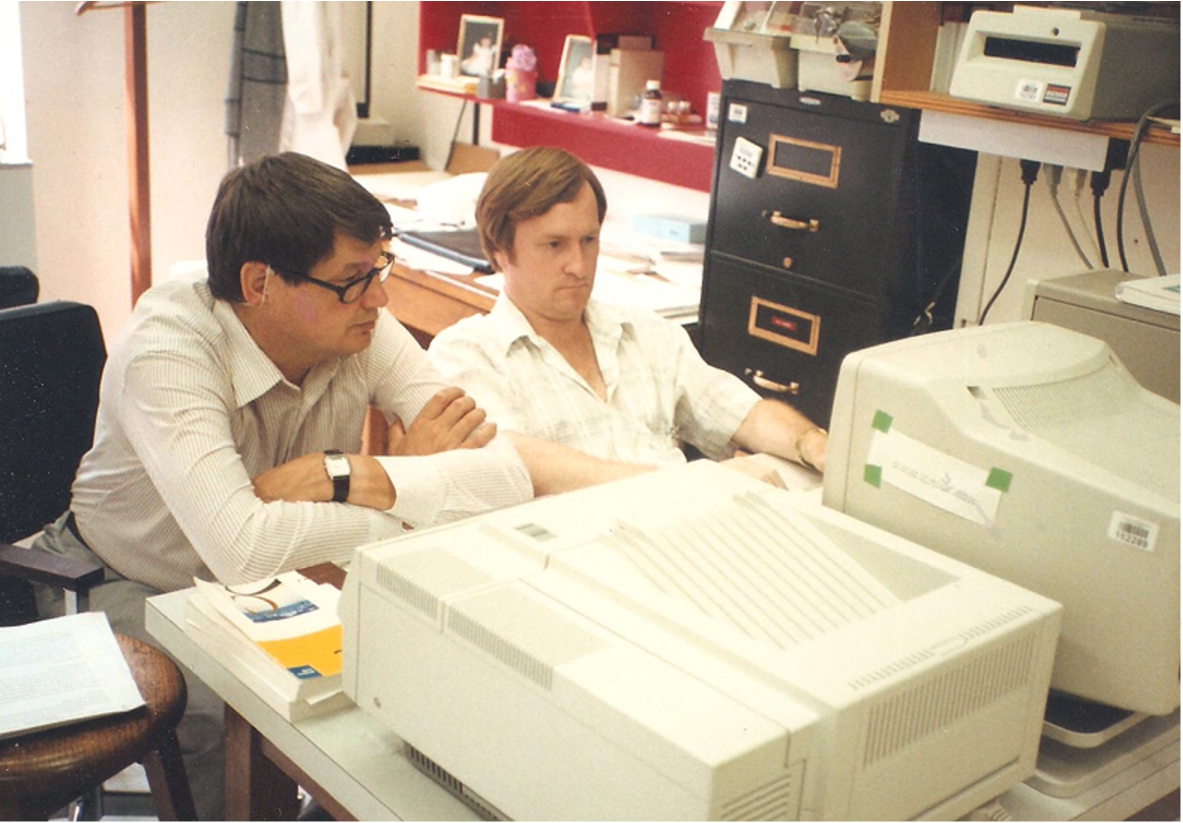
Using the FSD technique to analyse the infrared spectra of a variety of lipids and lipid membranes was not without its sceptics. I remember a meeting in the early 1980s where Gus Zerbi from Milan stood up and shouted: “We might be friends Henry, but I don’t believe a thing of what you just showed, you cannot achieve true resolution-enhancement through your FSD”. Technically speaking Zerbi was right and it took us some time to convince him and others that referring to this procedure as resolution-enhancement was incorrect and even misleading, but there was no mistaking that the effects of band narrowing were real. A classic example came from the analysis of lipid spectra when, after band narrowing by FSD, the broad CH2 scissoring band centered at 1467 cm−1 magically split into two distinct component bands at 1472 and 1462 cm−1. This exposed a typical factor group splitting of 10 cm−1 revealing an orthorhombic packing pattern of the acyl chains in the liquid-crystalline phase of these lipids. On the other hand when band narrowing by FSD could not split the band at 1467 cm−1 this revealed a hexagonal packing pattern of the acyl chains in those particular lipids. In subsequent years we made a lot of hay, providing insights into the structure and packing patterns of many types of lipids [8].
This brings me to our struggle for legitimacy and acceptance as bona fide vibrational spectroscopists. In 1983 when we proudly published a paper in Science [6] showing that it was possible to monitor the temperature-induced phase transition in the membranes of live bacteria, some of our colleagues from the Division of Physics accused us openly of “having sacrificed vibrational spectroscopy on the altar of biology”. Obviously to some staunch physicists the world of biology was simply too complex to be investigated by vibrational spectroscopy, an opinion shared to this day by some physicists. Fortunately Herzberg came to our rescue reminding his colleagues that vibrational spectroscopy does not belong exclusively to physicists, recognizing the nature of spectroscopy as an enabling technique and welcoming its application in other areas of human activity.
The next category of biomolecules we turned our attention to were the proteins. Attempts to use infrared spectra to determine the protein secondary structure go back a long way. The most promising feature in the IR spectrum of a protein that can be related to its secondary structure is a band in the region 1620–1680 cm−1 commonly known as the amide I band. There are very few proteins with a single secondary structure and the majority of real-life proteins exhibit only a broad, complex band contour resulting from the overlap of the different secondary structures present in that protein. This turned out to be fortuitous for our FSD band-narrowing methodology and we immediately started assessing the infrared spectra of a variety of commercially available proteins for their secondary structures. This work attracted visiting scientists who came to us with their own special proteins, leading to many fruitful co-operations and a substantial number of joint publications. Unfortunately it did not take long until we were faced with a scenario that we had not anticipated or foreseen. Several over-enthusiastic individuals were using these routines incorrectly and published the artefacts to which such measurements are susceptible, claiming them as real bands. This tainted our reputation and compromised the use of these procedures, forcing us to counter by publishing a number of reports in which we drew attention to the vulnerabilities these band-narrowing routines are susceptible to [13,32,33].
While our group did not make substantial contributions to the vibrational spectroscopy of nucleic acids, there were many groups active in this research, notably those around Dick Lord in Boston and Eliane Taillandier in Paris. We also paid little attention to another class of biomolecules, the carbohydrates or sugars, but a very active group developed in the 1980s around Dieter Naumann at the Robert Koch Institute in Berlin, dedicated to the study of pathogenic bacteria.
Then, in the 1990s, we became hooked on applications in medicine, particularly after we moved to a new NRC establishment, the Institute for Biodiagnostics in Winnipeg, dedicated to the non-invasive diagnosis of disease using NMR and vibrational spectroscopic techniques. We began with the relatively easy ex-vivo analysis of body fluids and developed the vibrational spectroscopic analysis as an adjunct tool to clinical chemistry. Initially we stuck to such obvious specimens as serum and urine, but later we extended this reagent-free spectral analysis to other body fluids such as synovial fluid for the diagnosis of arthritic conditions [26] or amniotic fluid for determining fetal lung maturity [20].
The vibrational spectroscopic analysis of excised tissues, dubbed spectral histopathology, turned out to be much more difficult since healthy tissue can be normal or abnormal and there are also many confounding factors that have to be taken into consideration. A clear goal of this spectral histopathology or spectropathology was and still remains the early detection of cancerous changes. At this point I have to mention an initial publication from our lab in Ottawa pitching the infrared spectroscopic analysis of exfoliated human cervical cells as an alternative to the classical H&E staining histopathology widely known as Pap-test [36]. This early report from 1991 was received with much interest but also drew considerable criticism. Subsequently many reports were published in which the authors claim to reveal cancerous or precancerous changes in cervical smears and in other human tissues, using spectral histopathology. In this context I welcome a recent reassessment by Max Diem et al. [11] which extols the benefits of spectral histopathology and spectral cytology, while also addressing the many pitfalls one must be aware of with these vibrational spectroscopic approaches.
The use of vibrational spectroscopy in the medical sciences received a major boost from the introduction of vibrational microspectroscopy and the development of infrared and Raman spectroscopic imaging techniques. In the early, pre-focal plane era, our lab in Winnipeg had to rely on UMA-type microspectrometers for the labour-intensive sequential mapping of keratin pearls in squamous cell carcinoma [24]. This was a far cry from the present day focal plane based imaging techniques which provide quick spatial and molecular details. For combining microspectroscopy with synchrotron radiation (which we did not have at NRC) I remain indebted to Gwyn Williams, who helped one of my PhD students, Lin-P’ing Choo to perform a first study of Alzheimer’s diseased tissue by synchrotron FT-IR microspectroscopy using the National Synchrotron Light source at Brookhaven [9]. In the late 1990s a team around Mike Sowa in Winnipeg exploited near-IR based imaging for monitoring tissue viability, skin hydration, burns and determining surgical margins [29].
By the turn of the century biomedical vibrational spectroscopy was in full swing with many new research groups springing up all over the world (sometimes entire institutes such as that of Juergen Popp in Jena). Our spectroscopy group in Winnipeg was soon overtaken by these events turning us from performers into observers. By 2002 I felt that it was time for me to leave the “hands-on” spectroscopy to the younger generation so I joined the Diplomatic Service, spending the next ten years as Science Counsellor at the Canadian Embassy in Berlin and later as Senior Science Counsellor to the Department of Foreign Affairs in Ottawa. Nonetheless, I remained faithful to the dictum “once a spectroscopist-always a spectroscopist” and continued following the advances in vibrational spectroscopy and dreaming about its future in the medical sciences. The progress achieved in the past 15+ years in applying vibrational spectroscopy to medical diagnostics is well documented in a number of multi-author books [2,10,19,21–23,25,27,30] and further below I will also muse about its future.
During my 30+ years at NRC I had the privilege of benefitting from the proximity of a personality such as Gerhard Herzberg or GH as he was known to those close to him (see Fig. 6). Working in the Division of Chemistry rather than in the Division of Physics had the advantage of not having to directly report to GH or having him appraise my work for promotions. This allowed us to have a closer personal relationship and also to socialize away from the lab. GH had strong feelings for the human side of science and frequently reminded us that we are dealing not only with science but also with people. He often helped fellow scientists in trouble and demonstrated in person in front of the Soviet Embassy in Ottawa to demand Dr Sakharov’s release from exile.
Fig. 6.
The author in the inner sanctuary of Herzberg’s office in Ottawa reading mail from dissidents (personal photograph). (Colors are visible in the online version of the article; http://dx.doi.org/10.3233/BSI-150118.)
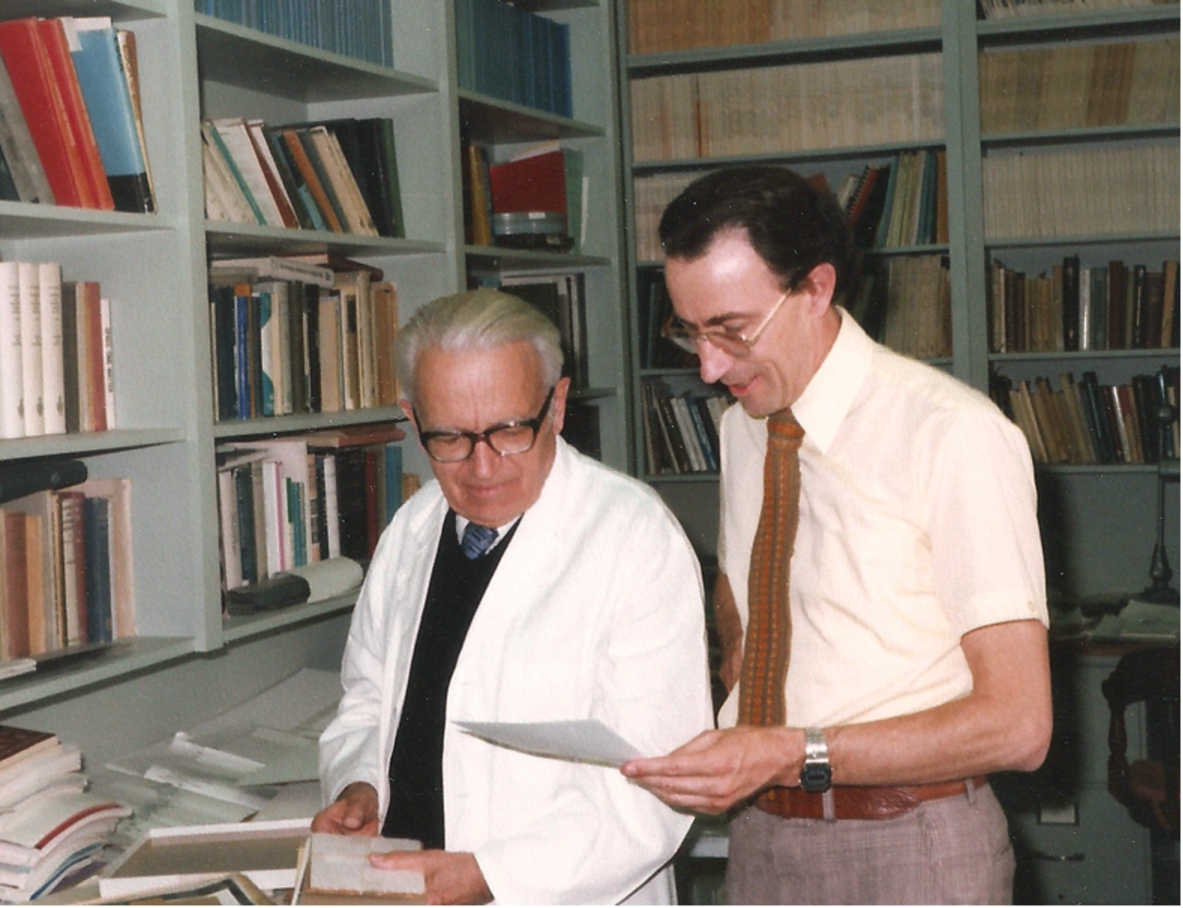
Herzberg himself was less interested in biology or medicine, but as a member of the American National Academy of Sciences he readily sponsored our work for publication in the prestigious proceedings, the PNAS. When he was already in his 80s GH attended a medical meeting in Washington titled Cancer Causation and Prevention: Biochemical Mechanisms, where he was invited to give a talk on oxygen and the electronic state found to be an important carrier of energy in cancer cells. His commentary was: “If anyone had suggested fifty years ago that my spectroscopic studies of oxygen would be important for cancer research I would have dismissed the idea off hand. But it shows that no one can foresee the applications of basic research”.
In concluding this part of my essay I want to comment on the evolution of conferences dedicated to vibrational spectroscopy in biology and medicine. My first attempts to connect with other like-minded spectroscopists occurred in the 1970s at the annual meetings of the American Biophysical Society which were always attended by some bio-spectroscopists. Then in 1983 a group of Canadians organised the NATO meeting entitled Advanced Study Institute on the Spectroscopy of Biological Molecules, held at a resort in Southern Italy. At this meeting we all decided to hold a follow-up meeting two years later, which took place in 1985 in Reims, France and became known as the first European Conference on the Spectroscopy of Biological Molecules (ECSBM), now a biennial meeting which continues to this day. Many present-day spectroscopists may recognize themselves in the photograph in Fig. 7 taken at the 4th ECSBM Conference in York. As the interest in biomedical applications of vibrational spectroscopy increased throughout the 1990s it was felt useful to have a conference dedicated solely to this topic. Hence we took advantage of the Centennial year 2000 to launch such a meeting for which we coined the catchy name “Shedding new Light on Disease: Optical Diagnostics for the New Millennium”. The conference was held at the Institute for Biodiagnostics in Winnipeg and became known under the acronym SPEC2000. This initial meeting also turned into a series of biennial conferences with the next one, SPEC2016, to be held in Montreal, thus returning to Canada where it all began. Notwithstanding these specialized conferences, biomedical applications of vibrational spectroscopy can be found today on the menu of many other conferences.
Fig. 7.
Group photograph from the 1991 ECSBM conference in York, UK (private photograph).
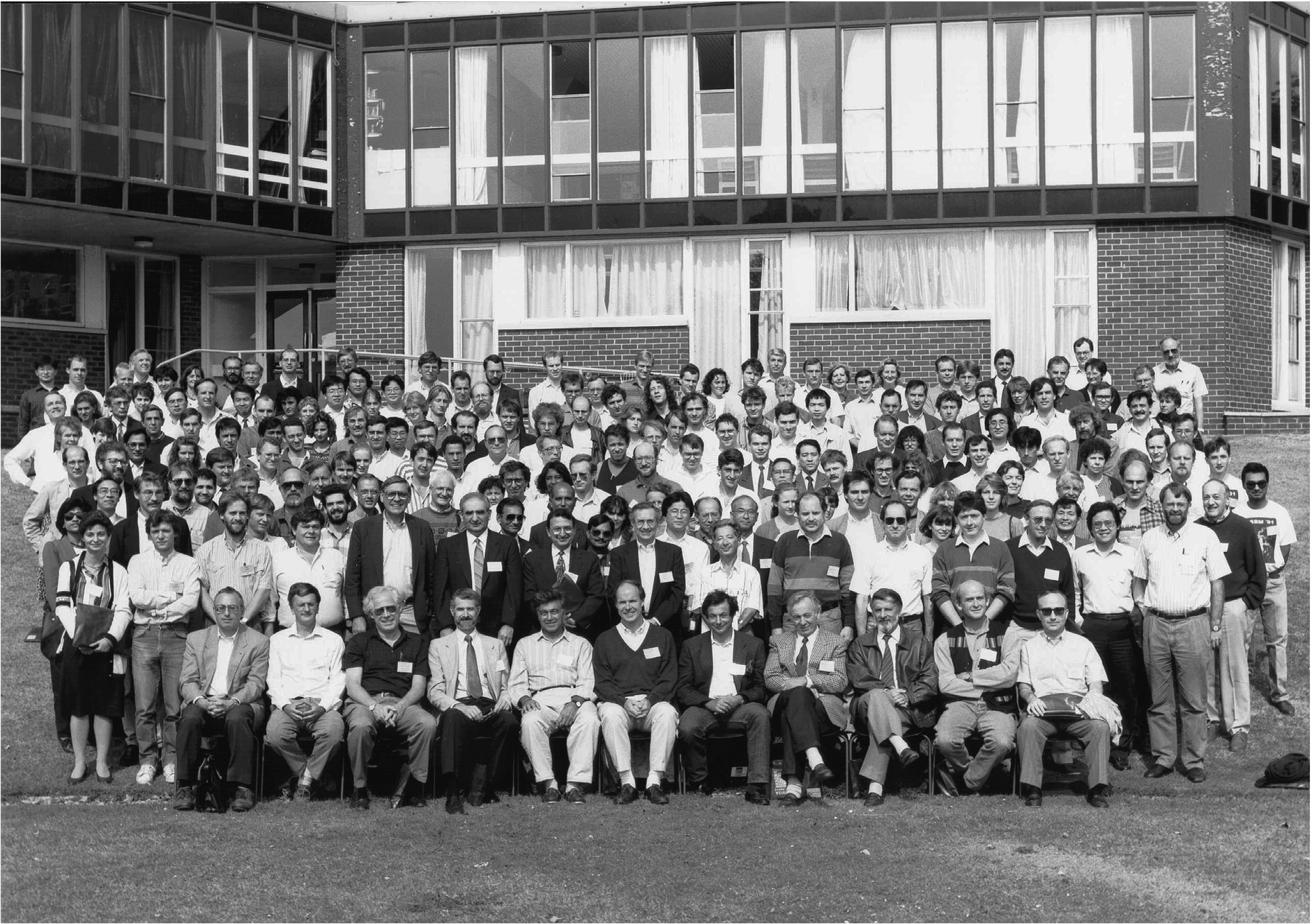
3.Musing on the future of biomedical vibrational spectroscopy: How afar will we go?
The evolution of biomedical vibrational spectroscopy should not be restricted solely to technological advances but ought to embrace also new ways of thinking. Today vibrational spectroscopy is solidly entrenched as a tool in medicine with applications ranging from the spectroscopy of body fluids, spectral cyto- and histopathology to the analysis of spectral imaging maps obtained from cells, tissues and even entire body parts. However something that has so far escaped exploration by vibrational spectroscopy is the human mind. And I do not mean the physical brain or the diseases that affect it such as Alzheimer’s, but I mean that attribute of us humans which controls our consciousness and self-awareness and makes each of us a unique individual. This clearly raises the question of the nature of the human mind, obviously a more philosophical question than a scientific one. Since philosophy deals with the contemplation of the unknown, it is not surprising that throughout the ages philosophers have wrestled with the question of the human mind and libraries the world over are filled with works on this subject. However, I believe it is imperative that science not concede the domain of the mind to philosophy only.
In keeping with my stated objective to speculate on forthcoming applications of vibrational spectroscopy in the medical sciences I now intend to focus on a vibrational spectroscopic approach to the human mind. My personal interest in this topic was stimulated not by a philosopher but by a scientist, and none other than Werner Heisenberg. In 1965 when I arrived in Munich as a young postdoctoral Humboldt Fellow, Heisenberg was the long standing president of the Alexander von Humboldt Foundation, a role which he took seriously and not in name only (see Fig. 8). Like many other great physicists Heisenberg had become interested in metaphysics later in life. I have to admit that I relished reading his book Physics and Philosophy: The Revolution in Modern Science [12] and was particularly impressed by his statement therein: “We have to remember that what we observe is not nature itself, but nature exposed to our method of questioning”. Every so often Heisenberg convened small groups of Humboldt Fellows to debate interdisciplinary topics, a favourite one being the Mind–Body theme. These informal round-table chats, sometimes over pretzels and beer, were well attended by a dynamic mix of physical and social scientists, MDs and even theologians. Yet, while the debates were very stimulating, they always ended in even more questions… and of course in more uncertainty. Heisenberg was an ardent Platonist, using the German word Geist (best translated as spirit or mind) which he regarded as being separate from the physical body. After leaving Germany and plunging into my professional life in Canada, my recollections of these animating debates lay dormant and resurfaced only now, some 50 years later. Certainly half a century of progress in science, and more specifically in vibrational spectroscopy, should allow us to revisit this topic.
Fig. 8.
Heisenberg proudly presents his Humboldt family to the President of Germany at a reception in the German White House in Bonn in July 1966. Foreground, left to right: The author, an unknown female Humboldt Fellow, Heinrich Luebke (German President), Werner Heisenberg; Background: Other Humboldt Fellows, vintage 1966. Note that while Heisenberg and the President had dropped their champagne glasses when the photograph was taken, this inexperienced youngster is still holding on to his glass (personal photograph).
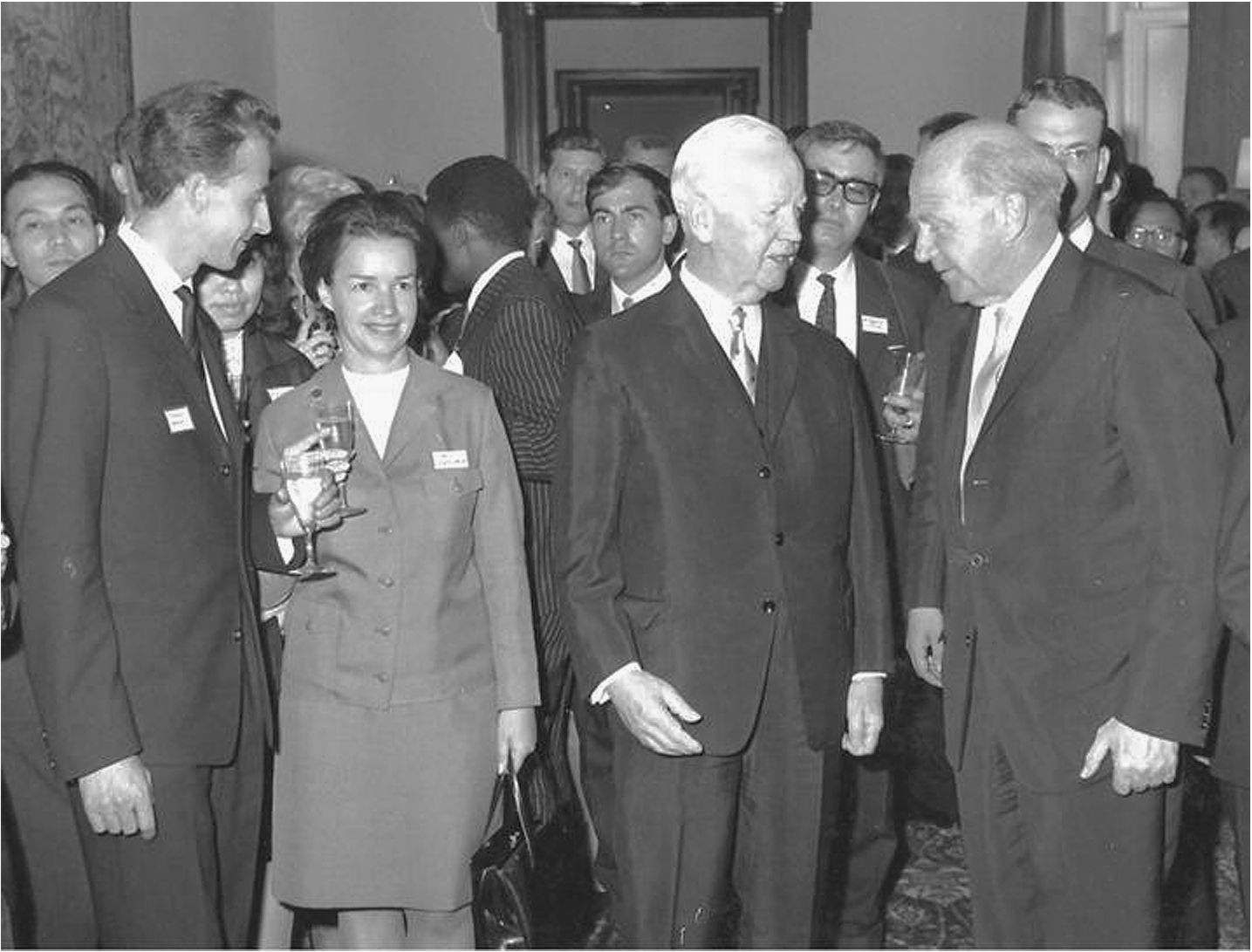
At this point we should review what is known about the human mind. The literature is full of anecdotal and circumstantial testimonies as to its nature however there is disappointingly few hard data or facts. Looking for the anatomical seat of the mind or the soul has certainly proven unsuccessful. Since artists find it easier to deal with abstract notions and constructs, in the visual arts the mind, spirit or soul is often depicted as a symbolic light that leaves the dying body. Many in the medical profession consider that the brain fully accounts for the mind and that the mind is simply a matter of brain function. I however prefer to go along with Plato, Heisenberg and many others, thinking about the human mind as an immaterial construct or process, involving some kind of electromagnetic or hitherto unknown form of energy which is able to interact with the physical brain (after all, physicists still do not know what dark energy is, although they readily admit it exists).
We certainly do know more about the physical brain. As a corporeal object the brain generates measurable output via EEG, the electroencephalographic potentials emitted by the brain, the so called brain waves. These electromagnetic waves, which occur in the frequency range below 100 Hz, are associated with specific mental arousal levels and a set of distinctive behavioural activities. It is not my intent to go into details here, as I am not a neuroscientist and certainly am not qualified to interpret EEGs or brain waves. However as a vibrational spectroscopist dealing regularly with electromagnetic waves, I cannot ignore the fact that brain waves have been shown to cause physiological effects. For instance, neuroscience experiments have shown that exposure to 12 Hz radiation stimulates the production of serotonin, a well-known mood elevation agent. With so many people taking antidepressant drugs today, would it be too far-fetched to contemplate the possibility of using molecular vibrations to diagnose and/or treat psychosomatic disorders or emotional brain diseases?
I suppose we all have experienced the sensation casually referred to as “feeling good vibrations” when encountering another human being. Is this only a figure of speech or could it suggest a relationship between our brain waves and the molecular vibrations so familiar to us spectroscopists? It might not be too unreasonable to assume that there exists a relationship between the physical brain and what we perceive as the non-physical mind, with the brain being the receiver and emitter, much like a radio or TV set. If we were to continue this analogy, then the mind could be thought of as representing the content of these audio or video messages. Certainly the air around us is filled with music and images which we cannot perceive unless they are detected and amplified by appropriate external devices. A similar thought-provoking analogy is that of the brain as a kind of universal transformer station which converts the energy of the mind to frequencies appropriate for us to use. Perhaps such analogies are oversimplified but they provide a good starting point for contemplating the brain–mind issue.
So let us return to the matter at hand, namely brain waves. From a pragmatic point of view the challenge lies in finding a link between brain waves, which occur in the range of 1–100 Hz and the molecular vibrations, which occur in the frequency range around 1012 Hz. Such a link is not unimaginable since both the brain waves and the molecular vibrations are part of the same electromagnetic spectrum, being separated by only about 40 octaves. Thus, if we were to take the brain wave at 12 Hz that is involved in the release of serotonin, we will find its 40th octave at 13.2 THz or 440 cm−1 which falls in the mid-IR/Raman range. What is needed at this point is a modality to convert brain waves into molecular vibrations and vice versa. To accomplish such a conversion, the waves or frequencies would have to be transduced 40 octaves up or down the continuum of the electromagnetic spectrum, something conceivable in theory, but not yet achieved in practice. By exploiting such bio-harmonic resonances we should then be able to detect brain waves in the vibrational spectrum (which would be of diagnostic value) and/or use discrete vibrational frequencies to affect and even manipulate brain waves for therapeutic purposes.
Obviously today we do not have the means to observe the 40th overtone of the brainwave frequency at 12 Hz and this will have to await the discovery of fundamentally new ways of detection and amplification. So while the vision of harnessing vibrational resonances in clinical neurophysiology might seem a dream today, we must not let present day limitations pose an insurmountable challenge to reaching for such frontiers. Indeed, over the years vibrational spectroscopy has been enriched by numerous innovations such as Resonance Raman-, Tip Enhanced Raman-, Stimulated Raman-, Surface Enhanced Raman/IR- Spectroscopy or Raman Optical Activity and Second Harmonic Generation, to name only a few, all of which employ tricks to overcome various limitations of conventional vibrational spectroscopy. Certainly there are abundant examples of former dreams becoming reality from those of Jules Verne to that of overcoming the diffraction limit of light as affirmed by the latest Nobel Prize in Chemistry.
4.Epilogue
Reflecting back on half a century of play in the sandbox labelled vibrational bio-spectroscopy I consider myself fortunate for having been around early in this game and then witnessing the consummation of the marriage between vibrational spectroscopy and medicine. My personal legacy is the path I have helped pave, the road to biomedical vibrational spectroscopy. I urge the young generation to use the spectroscopic toys handed down to them and also to dare stepping outside the sandbox to develop new ones. This author is convinced that vibrational spectroscopy and spectroscopic imaging have earned a permanent place in the medical toolbox of the future.
References
1 | [[1]] A. Barth and P. Haris, Infrared spectroscopy – Past and present, in: Biological and Biomedical Infrared Spectroscopy, P.I. Haris and A. Barth, eds, IOS Press, Amsterdam, (2009) , pp. 1–52. |
2 | [[2]] A. Barth and P.I. Haris (eds), Biological and Biomedical Infrared Spectroscopy, IOS Press, Amsterdam, (2009) , pp. 1–429. |
3 | [[3]] E.R. Blout and R.C. Mellors, Infrared spectra of tissues, Science 110: ((1949) ), 137–138. |
4 | [[4]] H.J. Byrne, K.M. Ostrowska, H. Nawaz, J. Dorney, A.D. Meade, F. Bonnier and F.M. Lyng, Vibrational spectroscopy: Disease diagnostics and beyond, in: Optical Spectroscopy and Computational Methods in Biology and Medicine, M. Baranska, ed., Springer, (2014) , pp. 355–399. |
5 | [[5]] D.G. Cameron and H.H. Mantsch, The phase transition of DPPC as seen by FT-IR difference spectroscopy, Biochem. Biophys. Res. Commun. 83: ((1978) ), 886–892. |
6 | [[6]] D.G. Cameron, A. Martin and H.H. Mantsch, Membrane isolation alters the gel to liquid crystal transition in live Acholeplasma laidlawii B, Science 219: ((1983) ), 180–182. |
7 | [[7]] P.R. Carey, Biochemical Applications of Raman and Resonance Raman Spectroscopies, Academic Press, New York, (1982) , pp. 1–262. |
8 | [[8]] H.L. Casal and H.H. Mantsch, Polymorphic phase behaviour of phospholipid membranes studied by infrared spectroscopy, Biochim. Biophys. Acta 779: ((1984) ), 381–401. |
9 | [[9]] L.P. Choo, D.L. Wetzel, W.C. Halliday, M. Jackson, S.M. Levine and H.H. Mantsch, In-situ characterization of beta-amyloid in Alzheimer’s diseased tissue by synchrotron Fourier transform infrared microspectroscopy, Biophysical J. 71: ((1996) ), 1672–1679. |
10 | [[10]] M. Diem, P.R. Griffiths and J.M. Chalmers (eds), Vibrational Spectroscopy for Medical Diagnosis, John Wiley & Sons, Chichester, (2008) , pp. 1–358. |
11 | [[11]] M. Diem, M. Miljkovic, B. Bird, T. Chernenko, J. Schubert, E. Marcsisin, A. Mazur, E. Kingston, E. Zuser, K. Papamarkakis and N. Laver, Applications of infrared and Raman microspectroscopy of cells and tissue in medical diagnostics: Present status and future promises, Spectroscopy: An International Journal 27: ((2012) ), 463–496. |
12 | [[12]] W. Heisenberg, Physics and Philosophy: The Revolution in Modern Science, Kindle Edition, (1958) , pp. 1–165. |
13 | [[13]] M. Jackson and H.H. Mantsch, The use and misuse of FT-IR spectroscopy in the determination of protein structure, Crit. Rev. Biochem. Mol. Biol. 30: ((1995) ), 95–120. |
14 | [[14]] R.N. Jones, Analytical applications of vibrational spectroscopy – A historical review, in: Chemical, Biological and Industrial Applications of Infrared Spectroscopy, J.R. Durig, ed., John Wiley & Sons, Chichester, (1985) , pp. 1–50. |
15 | [[15]] R.N. Jones, H.H. Mantsch and D.J. Moffatt, Computerized infrared spectroscopy: Beginnings and stops by the wayside, J. Mol. Structure 200: ((1989) ), 289–299. |
16 | [[16]] J.K. Kauppinen, D.J. Moffatt, D.G. Cameron and H.H. Mantsch, Noise in Fourier self-deconvolution, Applied Optics 20: ((1981) ), 1866–1879. |
17 | [[17]] J.K. Kauppinen, D.J. Moffatt, H.H. Mantsch and D.G. Cameron, Fourier-self-deconvolution: A method for resolving intrinsically overlapped bands, Applied Spectroscopy 35: ((1981) ), 271–276. |
18 | [[18]] J.K. Kauppinen, D.J. Moffatt, H.H. Mantsch and D.G. Cameron, Fourier transforms in the computation of self-deconvoluted and first-order derivative spectra of overlapped band contours, Analytical Chemistry 53: ((1981) ), 1454–1457. |
19 | [[19]] P. Lasch and J. Kneipp (eds), Biomedical Vibrational Spectroscopy, John Wiley & Sons, (2008) , pp. 1–385. |
20 | [[20]] K.Z. Liu, T.C. Dembinski and H.H. Mantsch, Rapid determination of fetal lung maturity from infrared spectra of amniotic fluid, American Journal of Obstetrics and Gynecology 178: ((1998) ), 234–241. |
21 | [[21]] D. Moss (ed.), Biomedical Applications of Synchrotron Infrared Microspectroscopy, RSC Publishing, Cambridge, (2011) , pp. 1–376. |
22 | [[22]] I. Rehman, Z. Movasaghi and S. Rehman (eds), Vibrational Spectroscopy for Tissue Analysis, CRC Press, Boca Raton, (2013) , pp. 1–305. |
23 | [[23]] R. Salzer and H.W. Siesler (eds), Infrared and Raman Spectroscopic Imaging, Wiley-VCH, Weinheim, (2009) , pp. 1–501. |
24 | [[24]] C.P. Schultz, K.Z. Liu, P.D. Kerr and H.H. Mantsch, In-situ infrared histopathology of keratinization in human oral/oropharyngeal squamous cell carcinoma, Oncology Research 10: ((1998) ), 277–286. |
25 | [[25]] F. Severcan and P.I. Haris (eds), Vibrational Spectroscopy in Diagnosis and Screening, IOS Press, Amsterdam, (2012) , pp. 1–421. |
26 | [[26]] R.A. Shaw, S. Kotowich, H.H. Eysel, M. Jackson, G.T. Thomson and H.H. Mantsch, Arthritis diagnosis based upon the infrared spectrum, Rheumatology International 15: ((1995) ), 159–165. |
27 | [[27]] F. Siebert and P. Hildebrandt, Vibrational Spectroscopy in Life Sciences, John Wiley & Sons, (2008) , pp. 1–320. |
28 | [[28]] I.C.P. Smith and H.H. Mantsch, A look at membranes by FT-NMR and FT-IR of deuterated lipids, Trends in Biochemical Sciences 4: ((1979) ), 152–154. |
29 | [[29]] M.G. Sowa, L. Leonardi, A. Matas, B.J. Schattka, M.D. Hewko, J.R. Payette and H.H. Mantsch, In-vivo tissue analysis by near-infrared spectroscopy, in: Encyclopedia of Analytical Chemistry, R.A. Meyers, ed., John Wiley & Sons, Chichester, (2000) , pp. 251–281. |
30 | [[30]] G. Srinivasan (ed.), Vibrational Spectroscopic Imaging for Biomedical Applications, McGraw-Hill, (2010) , pp. 1–350. |
31 | [[31]] S. Sunder, D. Cameron, H.H. Mantsch and H.J. Bernstein, Infrared and laser Raman studies of deuterated model membranes, Canad. J. Chem. 56: ((1978) ), 2121–2126. |
32 | [[32]] W.K. Surewics and H.H. Mantsch, New insight into protein secondary structure from resolution-enhanced infrared spectra, Biochim. Biophys. Acta 952: ((1988) ), 115–130. |
33 | [[33]] W.K. Surewics, H.H. Mantsch and D. Chapman, Determination of protein secondary structure by FT-IR spectroscopy, Biochemistry 32: ((1993) ), 389–394. |
34 | [[34]] D.L. Woernley, Infrared absorption curves for normal and neoplastic tissues and related biological substances, Cancer Research 12: ((1952) ), 516–523. |
35 | [[35]] P.T.T. Wong, D.J. Siminovitch and H.H. Mantsch, Structure and properties of model membranes: New knowledge from high-pressure vibrational spectroscopy, Biochim. Biophys. Acta 947: ((1988) ), 139–171. |
36 | [[36]] P.T.T. Wong, R.K. Wong, T.A. Caputo, T.A. Godwin and B. Rigas, Infrared spectroscopy of exfoliated human cervical cells: Evidence of extensive structural changes during carcinogenesis, Proc. Nat. Acad. Sci. 88: ((1991) ), 10988–10992. |