Hormetic effects by exercise on hippocampal neurogenesis with glucocorticoid signaling
Abstract
Exercise enhances adult hippocampal neurogenesis (AHN), although the exact nature of how this happens remains controversial. The beneficial effects of exercise vary depending upon the exercise condition, especially intensity. Most animal studies, however, have used wheel running, which only evaluates running distance (exercise volume) and does not consider intensity. In our rat model, we have found that exercise-induced neurogenesis varies depending on the intensity of the exercise and have found that exercise-enhanced neurogenesis is more pronounced with mild exercise than with moderate and/or intense exercise. This may be due, at least in part, to increased glucocorticoid (CORT) secretion. To test this hypothesis, we used our special exercise model in mice, with and without a stress response, based on the lactate threshold (LT) in which moderate exercise above the LT increases lactate and adrenocorticotropic hormone (ACTH) release, while mild exercise does not. Adult male C57BL/6J mice were subjected to two weeks of exercise training and AHN was measured with a 5-Bromo-2-deoxyuridine (BrdU) pre-injection and immunohistochemistry. The role of glucocorticoid signaling was examined using intrapertioneal injections of antagonists for the glucocorticoid receptor (GR), mifepristone, and the mineralocorticoid receptor (MR), spironolactone. We found that, while mild exercise increased AHN without elevating CORT blood levels, both MR and GR antagonists abolished mild-exercise-induced AHN, but did not affect AHN under intense exercise. This suggests a facilitative, permissive role of glucocorticoid and mineralocorticoid receptors in AHN during mild exercise (234/250)
Abbreviations
AHN | adult hippocampal neurogenesis |
LT | lactate threshold |
CORT | corticosterone |
MR | mineral corticoid receptor |
GR | glucocorticoid receptor |
BrdU | 5-Bromo-2-deoxyuridine |
DG | dentate gyrus |
Introduction
Within the hippocampus, a region of the brain that is associated with learning and memory, neurogenesis occurs in the dentate gyrus throughout the lifetime [1]. Exercise-induced adult hippocampal neurogenesis (AHN) has been widely reported in adult rodents that run on a voluntary running wheel or treadmill [2, 3]. However, the exact intensity of voluntary exercise (wheel running) is uncertain because it only allows for the evaluation of running distance. In our previous studies using rats, exercise intensity was defined using the blood lactate threshold (LT) during graded running exercises with use of a treadmill [4]. Using this model, we found that mild exercise enhances AHN, brain-derived neurotrophic factor (BDNF) and cognitive function, while intense exercise has less effect or even inhibits neurogenesis [5–7]. However, the mechanisms behind differences in AHN that are dependent on exercise intensity remain to be elucidated.
Exercise intensity influences glucocorticoid secretion. For instance, in humans, strongly elevated glucocorticoid levels are caused by intense exercise above the LT, an intensity at which blood lactate accumulation is accelerated, but not by exercise below the LT [8]. Similarly, in our rat model, mild exercise below the LT does not increase corticosterone (CORT), while moderate and intense exercise above the LT does lead to increased blood CORT levels [6]. Excess glucocorticoids are known to decrease the levels of hippocampal BDNF [9] and suppress neurogenesis [10]. Thus, the potential positive effects of intense exercise on AHN might be nullified with high blood CORT levels.
On the other hand, it has been reported that glucocorticoids can facilitate learning and memory in human subjects. In one study, the suppression of glucocorticoids with administration of metyrapone, a potent inhibitor of glucocorticoid synthesis, impaired cognitive function, but the impairment was reversed by glucocorticoid replacement [11]. In rats, a biphasic effect of glucocorticoids is reported for object recognition learning under conditions of emotional arousal [12]. Furthermore, adrenalectomy increased dentate gyrus neuronal apoptosis while a low dose of CORT blocked this effect [13] and a low dose CORT enhanced BDNF-TrkB signaling in the hippocampus [14], implying that glucocorticoids have biphasic effects on adult hippocampalneurogenesis.
The inverted U-shaped (“hormetic”[15, 16]) function of glucocorticoids is regulated by two kinds of receptors, the mineralocorticoid receptor (MR) and the glucocorticoid receptor (GR) [17]. For cell nuclear actions, these receptors have different affinities: MRs have a higher affinity for glucocorticoids than GRs in the hippocampus. Therefore, MRs are activated at low doses of CORT and GRs mainly become activated at high doses of CORT [18]. Yet, stress levels of glucocorticoids also activate non-genomic MR at the cell membrane and stimulate the release of glutamate [19]. Thus, low CORT would mainly activate nuclear MR and favor the inhibition of neuron apoptosis, while with a high dose, the activation of both non-genomic MR and GR occurs and, together with increased glutamate release, cause inhibition of neurogenesis in the dentate gyrus [20]. Indeed, some studies reported an inverted U-shaped effect of exercise as exercise hormesis effect [21, 22].
To study the role of glucorticoids in the exercise-hormesis effect, the exercise intensities for mice were defined as mild (sub-LT) and intense (supra-LT) and were used to study adult hippocampal neurogenesis (AHN). Possible involvement of glucocorticoid receptors in exercise-induced neurogenesis was investigated by administrating MR or GR antagonists. The results provide evidence that glucocorticoid receptor signaling enhances mild-exercise-enhanced AHN in the mouse hippocampus.
Methods
Animals
12-week-old male C57BL/6J mice (weight: 26–29 g; TLAS Co., Ltd., Tokyo, Japan) were maintained on a 12-h light/dark schedule (lights on at 7:00 a.m.) and given ad libitum access to food and water. All the experiments were performed in accordance to protocols approved by the University of Tsukuba Animal Experiment Laboratory Animals. Mice were acclimatized to ambient rearing conditions for seven days (3–5 mice per cage) and then, except operated mice, which were individually housed, all mice were group housed (3–5 mice per cage).
Exercise test to determine lactate threshold for mice
The mice (n = 8) were habituated to the treadmill apparatus (Natsume, Tokyo, Japan) for seven days of treadmill running at night. Animals ran on the treadmill once a day for a total of seven sessions in ten days. The running duration per session was 30 min, and treadmill speed was gradually increased from 5 to 30 m/min over the seven sessions. Increases in speed were implemented after the mice proved able to maintain their current exercise intensity. A shock grid at the rear of the treadmill gave a mild but aversive foot shock if the pace of the mice slowed below the treadmill rate. Very few shocks were administered during each training session. Some animals were excluded when they refused to run or, in rare cases, received an excessive foot shocks with immobility on the electric grid.
After running habituation, mice were anesthetized with pentobarbital sodium. The jugular vein was exposed with surgery, and a silicone catheter was inserted 1.3 mm into the vessel (Fig. 1A). The catheter was fixed in the vessel using silk thread and its distal end was fixed at the nape of the neck. After surgery, mice were kept individually in transparent polycarbonate cages for 3 days after surgery, and then, on the fourth days, the running tests to determine LT was done. The animals were allowed to rest on the treadmill for at least 15 min before the test. The initial treadmill velocity was 5 m/min and was increased by 2.5 m/min every 3 min until the point of exhaustion (Average 29.6 m/min). Blood samples were collected from the catheter 30 seconds before each speed increase to measure lactate for LT determination as per previous studies [4, 23]. After determining LT with the first experiment, we categorized treadmill speeds into supra-LT (30 m/min) and sub-LT (15 m/min).
Verification of sub-LT and supra-LT exercise intensity
To verify the exercise intensity, at least 2 days after the treadmill habituation period, the mice were divided into four groups, pre-exercise, control, sub-LT and supra-LT (n = 7, per group), and the mice were subjected to an exercise test (Fig. 2A). This test consisted of running at 30 or 15 m/min for 30 min. The control animals were placed on the treadmill without running for 30 min. After completion of the exercise test, the animals were immediately decapitated using a guillotine, and trunk blood was collected for blood lactate, glucose and plasma CORT measurements using an automated glucose-lactate analyzer (2300 Stat Plus, YSI, OH, USA) and radioimmunoassay.
Exercise training protocol for neurogenesis experiment
The exercise test revealed that the lactate threshold of mice, an index of exercise intensity, appeared at a running speed of approximately 20 m/min in the same way as it does for rats [24]. Thus, the running speeds in the exercise training protocol were classified as control (0 m/min), sub-LT (15 m/min) and supra-LT (30 m/min)(n = 8 per group). The mice were habituated to the treadmill apparatus for 5 min (KN-73, Natsume, Japan) before each training and then they were subjected to 30 min of treadmill exercise nearly daily (5 sessions/week for 2 weeks). The training of exercised mice included a gradual adaptation to the running. For instance, the sub-LT group ran at a speed of 10 m/min for the first 5 min and 15 m/min for the remaining 25 min, and the supra-LT group ran at a speed of 15 m/min for the first 2 min, 25 m/min for the next 3 min and 30 m/min for the remaining 25 min. Sedentary mice remained on the treadmill without running for the same amount of time (Fig. 3A).
Drug administration (glucocorticoid receptor antagonist and BrdU injection)
To block glucocorticoid receptors, mice received a subcutaneous injection of a selective GR antagonist, mifepristone (20 mg/kg/day, Sigma) [25], MR antagonist, spironolactone (12 mg/kg/day, Sigma), or Vehicle (polyethylene glycol, 5 ml/kg/day) (n = 6-7 per group) 1 hr prior to each treadmill training session. It has been reported that high doses of spironolactone decrease blood pressure. The dose that we administered for the present experiments would have no effect on blood pressure according to the previous reports [26]. To further assess neurogenesis, the mice were administrated intra-peritoneal injections of 5-Bromo-2-deoxyuridine (BrdU, 50 mg/kg/day, i.p.) 1 hr before each of the last five exercise sessions.
Sample collection
Two days after the last training session, the mice were deeply anesthetized with pentobarbital, and transcardially perfused with 0.9% saline. Brains were carefully removed and the hemispheres were separated. The right hemisphere was fixed overnight at 4°C with 4% paraformaldehyde in 0.1 M phosphate buffer and equilibrated in 30% sucrose. Sequential coronal sections (50μm thick) of the entire hippocampus were collected individually in 96-multiwell culture plates. One in eight random serial sections was collected for immunohistochemistry, and the brain slices were pre-incubated in PB with 1.0% Triton X-100 and 1.0% bovine serum albumin. To measure CORT levels, cardiac blood was collected before the heart was perfused with saline.
Immunohistochemical analysis
Triple immunofluorescence staining for BrdU, DCX, and NeuN was performed on one series of sections selected at random, as described previously [27]. Briefly, one-in-eight series of sections (50μm thick) were used for cell counting (7-8 sections per mouse). One series was randomly selected, and the sample was pretreated with 2 N HCl at 37°C for 30 min to denature the DNA. Then the free floating slices were incubated for 2 days at 4°C with the primary antibodies diluted with 0.1 M PB containing 1% bovine serum albumin (BSA) and 1% Triton X-100. Rat monoclonal anti-BrdU antibody (AbD Serotec, UK, 1:500), goat polyclonal anti-DCX (Santa Cruz, USA; 1:250), and mouse monoclonal anti-NeuN (Chemicon, USA; 1:500) were used as the primary antibodies. The slices were then incubated for 24 h at 4°C with an appropriate secondary antibody: Cy3 donkey anti-rat (JACKSON, USA; 1:500), Alexa Fluor 488 donkey anti-goat (Invitrogen, USA; 1:500), or AMCA donkey anti-mouse (JACSON, USA; 1:250). The sections were mounted on gelatin-covered slides and analyzed with a Leica DMRB optical microscope (Leica, Bensheim, Germany). To estimate the total number of target cells in whole hippocampus, first, the immunolabeled cells in the subgranular zone (SGZ) and the granule cell layer (GCL) of the DG were counted by the hand. Based on this number, the cell density in each section was calculated by multiplication of the DG area by the cell number in the section. The DG area was made visible with Nissl staining, and quantifiedusing the image analysis software Image J ver. 1.44 (NIH, http://rsb.info.nih.gov/ij). Finally, the total number of labelled cells was calculated by multiplication of the mean cell density in all sections by the totalDG volume.
Measurement of plasma corticosterone
Plasma CORT levels were measured with radioimmunoassay using competitive binding as described previously [28]. Briefly, we utilized [3H]-CORT (Tokyo Chemical Industry) and anti-CORT antibody (Cosmo Bio). After 16 hours, anti-CORT bound CORT was separated from unbound CORT by adding 500μl of Dextran-coated charcoal. The samples were centrifuged at 4°C (3,000 rpm, 10 min) and immediately transferred to scintillation vials filled with 4 ml of a liquid scintillation cocktail (Clear-sol II; Nacalai Tesque). The vials were shaken followed by a count of the CORT concentration with a LS6000 Beckman scintillation counter (Beckman Coulter). The detection limit of the CORT assay was 0.2 ng/dl. Final plasma CORT concentration was determined by the average of its duplicate concentrations.
Statistical analysis
Data are expressed as mean ± S.E.M. The results were analyzed with modified regression analysis or one-way ANOVA followed by Tukey’s post hoc tests or two-way ANOVA followed by Bonferroni post hoc tests whenever appropriate. Significance was established at p < 0.05. Sample sizes are indicated by n.
Results
LT determination for mice during the exercise test
The experimental model for determining the lactate threshold (LT) in mice during the exercise test is shown in Fig. 1. Lactate concentrations in the blood taken through the jugular catheter were measured every 3 min while increasing the treadmill speed. The LT was found at speeds of 17.5∼20 m/min (Fig. 1B), which is equal to that for male rats.
Blood lactate, glucose, and plasma corticosterone concentration after different intensities of exercise
To evaluate changes in the physiological index after different intensities of exercise, we conducted a test that consisted of running at 15 (sub-LT) or 30 (supra-LT) m/min on a level treadmill for 30 min (Fig. 2A). Blood lactate levels were significantly elevated in the supra-LT group compared to the sub-LT and control groups (F (3,24) = 23.47, p < 0.0001; control vs supra-LT, sub-LT vs supra-LT, p < 0.0001) (Fig. 2B). Plasma CORT concentrations were significantly elevated in the supra-LT mice compared to the control mice (F (3,24) = 6.31, p = 0.0026; control vs supra-LT, p < 0.01) (Fig. 2C). There were no significant changes in the blood glucose levels among these groups (Fig. 2D). These results reveal that exercise in the supra-LT (30 m/min) group was quite stressful, while that in the sub-LT (15 m/min) group was stress free.
Effect of treadmill running at different intensities on adult hippocampal neurogenesis
The total numbers of BrdU+ cells in the control, sub-LT, and supra-LT groups were 2764 ± 200, 3404 ± 161 and 2883 ± 272, respectively. BrdU+ cells were significantly increased in the sub-LT group while the supra-LT group showed no significant difference compared to the control group (F (2,21) = 3.65, p = 0.044; control vs sub-LT, p < 0.05) (Fig. 3C). The numbers of BrdU+/DCX+ double-labeled cells in the control, sub-LT, and supra-LT groups were 1290 ± 109, 1969 ± 104 and 1417 ± 118, respectively. BrdU+/DCX+ labeled cells of the sub-LT exercise groups were significantly increased compared to those of the control and supra-LT groups (F (2,21) = 10.69, p = 0.0006; control vs sub-LT, p < 0.001, sub-LT vs supra-LT, p < 0.01) (Fig. 3D). In addition, sub-LT exercise significantly enhanced BrdU+/NeuN+ labeled cells (F (2,21) = 4.69, p = 0.02; control vs sub-LT, p < 0.05). The numbers of BrdU+/NeuN+ double-labeled cells in the control, sub-LT, and supra-LT groups were 120 ± 20, 219 ± 25 and 154 ± 25, respectively (Fig. 3E). These results suggest that mild exercise (sub-LT), but not intensive exercise (supra-LT), may enhance adult neurogenesis in the mouse dentate gyrus.
Effect of glucocorticoid signaling on exercise-induced neurogenesis
The role of glucocorticoid signaling was evaluated by the daily injection of the MR antagonist spironolactone, or the GR antagonist, mifepristone. The adrenal weight and adrenal to body weight ratio were significantly increased with supra-LT exercise when mifepristone or spironolactone was applied (p < 0.05, p < 0.01 in comparison to respective control mice, Table1). Although the serum CORT levels, assessed two days after the last training session, did not change significantly in the antagonist-treated groups, similarly for the results of adrenal weight (Table 1). For sub-LT exercise, there was no effect on plasma CORT (Fig. 2) or body or adrenal weights or adrenal to body weight ratio (Table 1). Much to our surprise, both MR and GR glucocorticoid antagonists blocked the enhancing effects of sub-LT exercise on BrdU+, BrdU+/DCX+ or BrdU+/NeuN+ labeled cells, while no significant changes between the vehicle control and the antagonist-treated control groups were observed (Fig. 4A, B & C). These results suggest that mild-exercise-induced neurogenesis may normally be facilitated by CORT acting permissively via MR and GR.
Discussion
To investigate role of adrenal steroids in exercise-induced AHN, in this study, we defined the exercise intensity for mice based on the LT, and we verified the effects of different exercise intensities on AHN and the involvement of glucocorticoid signaling. We report that mild exercise (sub-LT), which does not elevate blood lactate and CORT, enhances adult hippocampal neurogenesis in mice. Surprisingly, glucocorticoid signaling in this mild-exercise-induced neurogenesis plays a positive, permissive role and enhances neurogenesis via MR and GR.
There are many studies demonstrating that exercise leads to increase AHN [29], but our report is the first to demonstrate that adult neurogenesis in the mouse could be differentially regulated under different exercise intensities as defined by the LT. In work using a running wheel exercise distance is the only measurable parameter. In rats, we established an exercise model using treadmill running to define mild and high intensities of exercise in reference to their LT ( 20 m/min), a physiologic index of exercise intensity as was previously reported [4]. Using this model in the rat, we found that mild exercise below the LT enhances AHN and spatial learning memory [5, 7]. Our present study thus established for mice an LT model for investigative the physiological and neurobiological effect of exercise intensity as well as duration and extend to mice our previous finding in rats showing that mild exercise enhances hippocampal plasticity.
We used both MR and GR antagonists (mifepristone for GR; spironolactone for MR) to test the possible role of glucocorticoid signaling in the underlying mechanisms of exercise-induced neurogenesis. The positive, apparently permissive, involvement of MR and GR in mild-exercise-enhanced neurogenesis is a surprising finding of this study that is consistent with another report that glucocorticoids are required for the voluntary-exercise-induced enhancement of learning and memory [30]. It has been shown that MR is expressed in newborn neurons in the dentate gyrus [31] and that MR activation inhibits the apoptosis of hippocampal granule cells [32, 33]. Besides preventing apoptosis, there are indications that aldosterone, via MR, can increase neurogenesis in the dentate gyrus of adrenalectomized rats [34]. This is consistent with our finding that spironolactone inhibits exercise-induced neurogenesis. As MR is also expressed in mature granule cells as well as dividing cells, MR might also be involved with exercise-induced neurogenesis in the maintenance of the survival of dentate gyrus granule neurons [31].
Moderate activation of GR exerts positive effects on the brain [35]. Furthermore, other mediators such as oxytocin [36] and BDNF could work synergistically with glucocorticoids on AHN. For BDNF, several studies have reported ligand-independent activation by CORT of the BDNF receptor TrkB (Tropomyosin-related kinase B), which promotes BDNF-triggered downstream signaling [37, 38]. Moreover, a down-regulation of hippocampal BDNF expression and a reduction of hippocampal neurogenesis have been reported in GR-heterozygous mutant mice (GR ± ) with a 50% GR gene reduction [39]. Therefore, as shown in our study, it is possible that GR participates in a positive, permissive way with other mediators in the enhancement of neurogenesis.
In general, moderate chronic exercise is reputed to be optimum for maintaining health, while severe exercise can cause negative stress effects on the brain [6]. In this report, because excessive glucocorticoids are known to decrease ANH via glucocorticoid receptors [40], we postulated that, in the supra-LT group with stress, high glucocorticoid levels would inhibit the beneficial exercise effect on AHN. However, our present results in mice showed that, while intense exercise did not increased AHN even after the administrated of GR or MR antagonists. This was unexpected since increased levels of glucocorticoids suppress AHN, and the removal of glucocorticoid by adrenalectomy and the blockage glucocorticoid receptors by antagonist reverses this effect [41, 40]. Yet, glucocorticoids are not necessarily involved in the inhibition of AHN by stress; for example, Muller et al. [42] reported the inhibitory effects on AHN by sleep deprivation stress independent of glucocorticoids. In an exercise stress model, other factors, such as IL-6 and TNF-α, which dampen AHN [43, 44] should be considered.
In conclusion, our results demonstrate that mild exercise below the LT enhances adult hippocampal neurogenesis and this effect can be nullified by MR or GR antagonist administration, suggesting that glucocorticoid signaling may play a permissive facilitative role, along with other mediators, in mild-exercise-induced neurogenesis.
ACKNOWLEDGMENTS
This study was supported in part by Grant-in-Aid for Scientific Research (B) of the Japan Society for the Promotion of Science (no. 20300214), by the Ministry of Education, Culture, Sports, Science and Technology (MEXT) for the Body and Mind Integrated Sports Sciences Project (2010) and by the 21st Century Center of Excellence program from the Ministry of Education, Culture, Sports and Technology of Japan. Masahiro Okamoto and Takashi Matsui are both Research Fellows of the Japan Society for the Promotion of Science.
REFERENCES
1 | Gage FH(2000) Mammalian neural stem cellsScience287: 14331438 |
2 | Van Praag H, Christie BR, Sejnowski TJ, Gage FH(1999) Running enhances neurogenesis, learning, and long-term potentiation in miceProc Natl Acad Sci U S A96: 231342713431 |
3 | Ra SM, Kim H, Jang MH, Shin MC, Lee TH, Lim BV, Kim CJ, Kim EH, Kim KM, Kim SS(2002) Treadmill running and swimming increase cell proliferation in the hippocampal dentate gyrus of ratsNeurosci Lett333: 123126 |
4 | Soya H, Mukai A, Deocaris CC, Ohiwa N, Chang H, Nishijima T, Fujikawa T, Togashi K, Saito T(2007) Threshold-like pattern of neuronalactivation in the hypothalamus during treadmill running:Establishment of a minimum running stress (MRS) rat modelNeurosci Res58: 341348 |
5 | Okamoto M, Hojo Y, Inoue K, Matsui T, Kawato S, McEwen BS, Soya H(2012) Mild exercise increases dihydrotestosterone in hippocampus providing evidence for androgenic mediation of neurogenesisProc Natl Acad Sci109: 1310013105 |
6 | Soya H, Nakamura T, Deocaris CC, Kimpara A, Iimura M, Fujikawa T, Chang H, McEwen BS, Nishijima T(2007) BDNF induction with mild exercisein the rat hippocampusBiochem Biophys Res Commun358: 961967 |
7 | Inoue K, Hanaoka Y, Nishijima T, Okamoto M, Chang H, Saito T, Soya H, Sciences SLong-term Mild Exercise Training Enhances Hippocampus-dependent Memory in Rats |
8 | Farrell PA, Garthwaite TL, Gustafson AB(1983) Plasma adrenocorticotropin and cortisol responses to submaximal and exhaustive exerciseJ Appl Physiol55: 514411444 |
9 | Schaaf MJM, De Jong J, De Kloet ER, Vreugdenhil E(1998) Downregulation of BDNF mRNA and protein in the rat hippocampusby corticosteroneBrain Res813: 112120 |
10 | Gould E, Cameron HA, Daniels DC, Woolley CS, McEwen BS(1992) Adrenal hormones suppress cell division in the adult rat dentate gyrusJ Neurosci12: September36423650 |
11 | Lupien SJ, Wilkinson CW, Brière S, Ménard C, Ng Ying Kin NMK, Nair NPV(2002) The modulatory effects of corticosteroids on cognition: Studies in young human populationsPsychoneuroendocrinology27: 401416 |
12 | Okuda S, Roozendaal B, Mcgaugh JL(2004) Glucocorticoid effects on object recognition memory require training-associated emotional arousal101: 3853858 |
13 | Sloviter RS, Valiquette G, Abrams GM, Ronk EC, Sollas AL, Paul LA, Neubort S(1989) Selective loss of hippocampal granule cells in the mature rat brain after adrenalectomyScience243: 535538 |
14 | Schaaf MJM, Hoetelmans RWM, De Kloet ER, Vreugdenhil E(1997) Corticosterone regulates expression of BDNF and trkB but not NT-3 and trkC mRNA in the rat hippocampusJ Neurosci Res48: 334341 |
15 | Lupien S, Mcewen B(1997) The acute effects of corticosteroids on cognition: Integration of animal and human model studiesBrain Res Rev24: 1127 |
16 | Lupien SJ, Buss C, Schramek TE, Maheu F, Pruessner J(2005) Hormetic influence of glucocorticoids on human memoryNonlinearity Biol Toxicol Med3: 12356 |
17 | De Kloet ER, Vreugdenhil E, Oitzl MS, Joëls M(1998) Brain corticosteroid receptor balance in health and diseaseEndocr Rev19: 269301 |
18 | Reul JMHM, De Kloet ER(1985) Two receptor systems for corticosterone in rat brain: Microdistribution and differential occupationEndocrinology117: 25052511 |
19 | Karst H, Berger S, Turiault M, Tronche F, Schütz G, Joëls M(2005) Mineralocorticoid receptors are indispensable for nongenomicmodulation of hippocampal glutamate transmission bycorticosteroneProc Natl Acad Sci U S A102: 521920419207 |
20 | Cameron HA, McEwen BS, Gould E(1995) Regulation of adult neurogenesisby excitatory input and NMDA receptor activation in the dentategyrusJ Neurosci15: 646874692 |
21 | Goto S, Naito H, Kaneko T, Chung HY, Radák Z(2007) Hormetic effects of regular exercise in aging: Correlation with oxidative stressAppl Physiol Nutr Metab32: 5948953 |
22 | Radak Z, Chung HY, Goto S(2005) Exercise and hormesis: Oxidative stress-related adaptation for successful agingBiogerontology6: 17175 |
23 | Beaver WL, Wasserman K, Whipp BJ(1985) Improved detection of lactate threshold during exercise using a log-log transformationJ Appl Physiol59: 19361940 |
24 | Saito T, Soya H(2004) Delineation of responsive AVP-containing neurons to running stress in the hypothalamusAm J Physiol Regul Integr Comp Physiol286: 484490 |
25 | Mailliet F, Qi H, Rocher C, Spedding M, Svenningsson P, Jay TM(2008) Protection of stress-induced impairment of hippocampal/prefrontal LTP through blockade of glucocorticoid receptors. Implication of MEK signalingExp Neurol211: 593596 |
26 | Oyamada N, Sone M, Miyashita K, Park K, Taura D, Inuzuka M, Sonoyama T, Tsujimoto H, Fukunaga Y, Tamura N, Itoh H, Nakao K(2008) The role of mineralocorticoid receptor expression in brain remodeling after cerebral ischemiaEndocrinology149: 3777 |
27 | Llorens-Martín M, Torres-Alemán I, Trejo JL(2006) Pronounced individual variation in the response to the stimulatory action of exercise on immature hippocampal neuronsHippocampus16: 480490 |
28 | Kawashima H, Saito T, Yoshizato H, Fujikawa T, Sato Y, McEwen BS, Soya H(2004) Endurance treadmill training in rats alters CRH activity in the hypothalamic paraventricular nucleus at rest and during acute running according to its periodLife Sci76: 7763774 |
29 | Voss MW, Vivar C, Kramer AF, Van Praag H(2013) Bridging animal and human models of exercise-induced brain plasticityTrends Cogn Sci17: 10525544 |
30 | Hajisoltani R, Rashidy-Pour A, Vafaei AA, Ghaderdoost B, Bandegi AR, Motamedi F(2011) The glucocorticoid system is required for thevoluntary exercise-induced enhancement of learning and memory inratsBehav Brain Res219: 7581 |
31 | Garcia A, Steiner B, Kronenberg G, Bick-Sander A, Kempermann G(2004) Age-dependent expression of glucocorti-coid-and mineralocorticoid receptors on neural precursor cellpopulations in the adult murine hippocampusAging Cell3: 363371 |
32 | Gould E, Woolley CS, McEwen BS(1990) Short-termglucocorticoid manipulations affect neuronal morphology andsurvival in the adult dentate gyrusNeuroscience37: 367375 |
33 | Woolley CS, Gould E, Sakai RR, Spencer RL, McEwen BS(1991) Effects of aldosterone or RU2 treatment on adrenalectomy-induced cell death in the dentate gyrus of the adult ratBrain Res554: 312315 |
34 | Fischer AK, Von Rosenstiel P, Fuchs E, Goula D, Almeida OFX, Czéh B(2002) The prototypic mineralocorticoidreceptoragonist aldosterone influences neurogenesis inthe dentate gyrus of the adrenalectomizedratBrain Res947: 290293 |
35 | Diamond DM, Bennett MC, Fleshner M, Rose GM(1992) Inverted-Urelationship between the level of peripheral corticosterone andthe magnitude of hippocampal primed burst potentiationHippocampus2: 421430 |
36 | Leuner B, Caponiti JM, Gould E(2012) Oxytocin stimulates adult neurogenesis even under conditions of stress and elevated glucocorticoidsHippocampus22: 861868 |
37 | Jeanneteau F, Garabedian MJ, Chao MV(2008) Activation of Trkneurotrophin receptors by glucocorticoids provides aneuroprotective effectProc Natl Acad Sci U S A105: 48624867 |
38 | Numakawa T, Kumamaru E, Adachi N, Yagasaki Y, Izumi A, Kunugi H(2009) Glucocorticoid receptor interaction with TrkB promotes BDNF-triggered PLC-gamma signaling for glutamate release via a glutamate transporterProc Natl Acad Sci U S A106: 647652 |
39 | Kronenberg G, Kirste I, Inta D, Chourbaji S, Heuser I, Endres M, Gass P(2009) Reduced hippocampal neurogenesis in the GR ± genetic mouse model of depressionEur Arch Psychiatry Clin Neurosci259: 499504 |
40 | Wong EYH, Herbert J(2004) Roles of mineralocorticoid and glucocorticoidreceptors in the regulation of progenitor proliferation in theadult hippocampusEur J Neurosci22: September 2004785792 |
41 | Cameron HA, Gould E(1994) Adult neurogenesis is regulated by adrenal steroids in the dentate gyrusNeuroscience61: 203209 |
42 | Mueller AD, Pollock MS, Lieblich SE, Epp JR, Galea LAM, Mistlberger RE(2008) Sleep deprivation can inhibit adult hippocampal neurogenesis independent of adrenal stress hormonesAm J Physiol Regul Integr Comp Physiol294: R1693R1703 |
43 | Vallières L, Campbell IL, Gage FH, Sawchenko PE(2002) Reduced hippocampal neurogenesis in adult transgenic mice with chronic astrocytic production of interleukin-6J Neurosci22: 2486492 |
44 | Iosif RE, Ekdahl CT, Ahlenius H, Pronk CJH, Bonde S, Kokaia Z, Jacobsen S-EW, Lindvall O(2006) Tumor necrosis factor receptor 1 is a negative regulator of progenitor proliferation in adult hippocampal neurogenesisJ Neurosci26: 3897039712 |
Figures and Tables
Fig.1
A typical lactate threshold (LT) profile for a mouse during a gradual increase of running speed protocol (0–35 m/min) (A) To collect blood during treadmill running, a silicone catheter was inserted through the jugular vein to the right atrium three days before the graded exercise test. (B) Typical data for LT of one mouse (No. 4) is shown. The LT (around 20 m/min) was determined from the non-linear increase in blood lactate levels vs running speed using a modified regression analysis. Inset is average LT for eight mice.
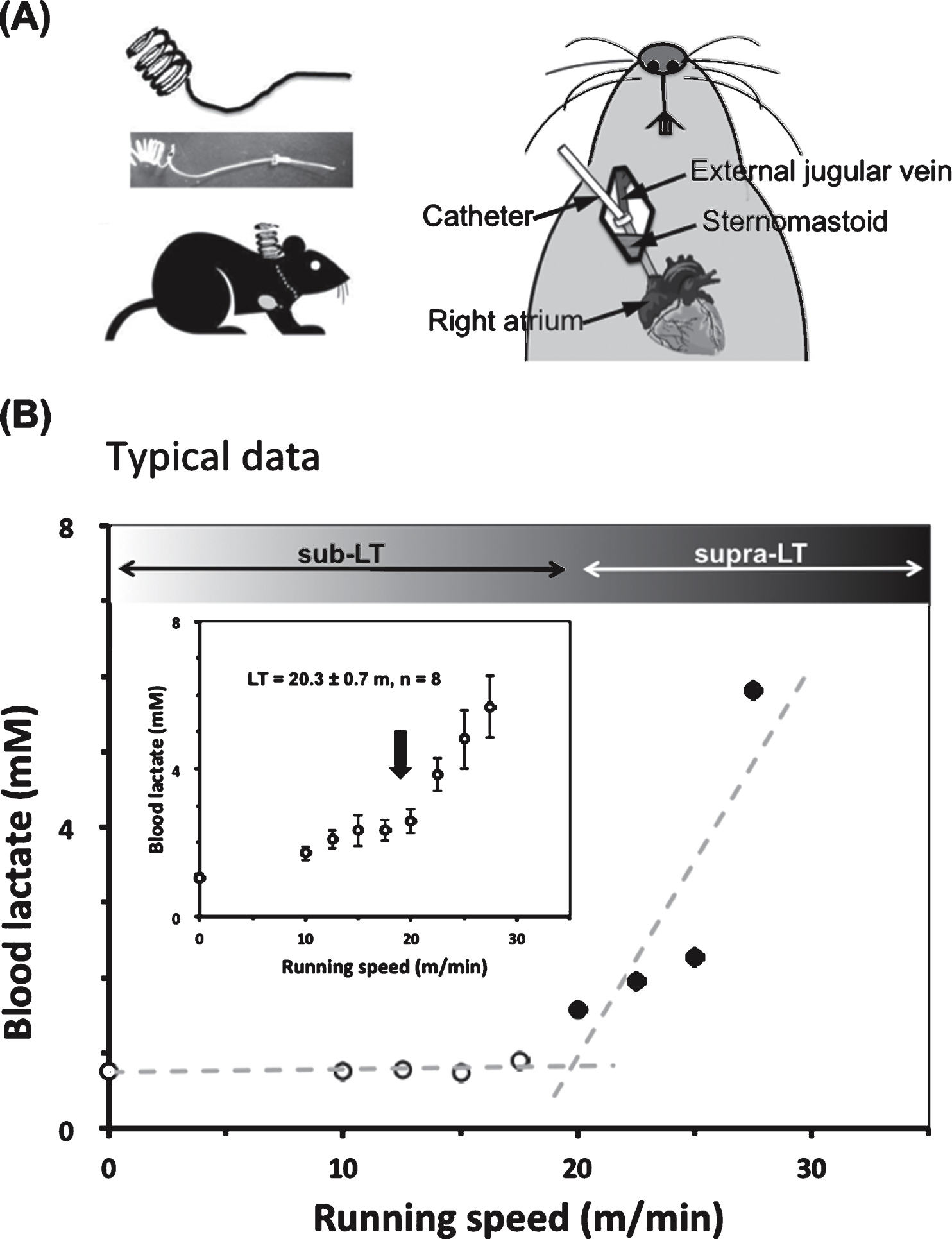
Fig.2
Determination of blood lactate, glucose, and plasma corticosterone after different intensities of exercise (A) Protocols for different intensities of exercise, (B) blood lactate levels (F (3,24) = 23.47, p < 0.0001), (C) plasma corticosterone levels (F (3,24) = 6.31, p = 0.0026), and (D) blood glucose levels (F (3,24) = 1.17, p = 0.34). Data represent the mean ± SEM (n = 7 mice per group). **, p < 0.01, ***, p < 0.001 in comparison with control and # # # , p < 0.001 in comparison to sub-LT (one-way ANOVA Tukey post hoc tests).
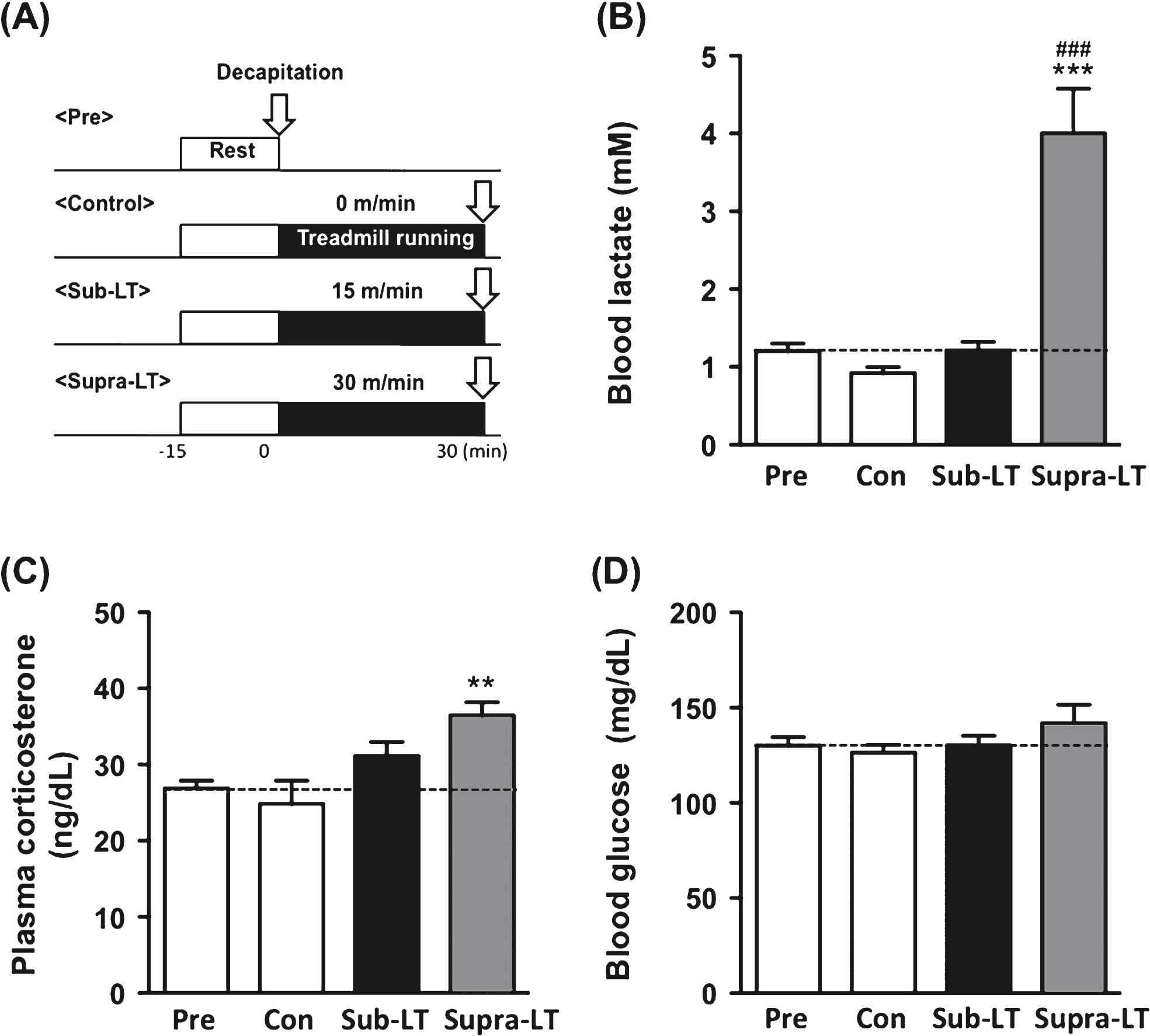
Fig.3
Effects of different exercise intensities on adult neurogenesis of male mice (A) Timeline of experiment, (B) A representative confocal micrograph of triple labeling of BrdU (red), the immature neuron marker DCX (green), and the mature neuron marker NeuN (blue) in the hippocampal dentate area. Scale bars, 50μm. (C) Total number of BrdU+ cells (F (2,21) = 3.65, p = 0.044). (D) Number of BrdU+/DCX+ cells (F (2,21) = 10.69, p = 0.0006). (E) Number of BrdU+/NeuN+ cells in the dentate gyrus (F (2,21) = 4.69, p = 0.02). Data represent the mean ± SEM (n = 8 mice per group). *, p < 0.05, ***, p < 0.001 in comparison to control mice and # # , p < 0.01 in comparison with supra-LT (one-way ANOVA Tukey post hoc tests).
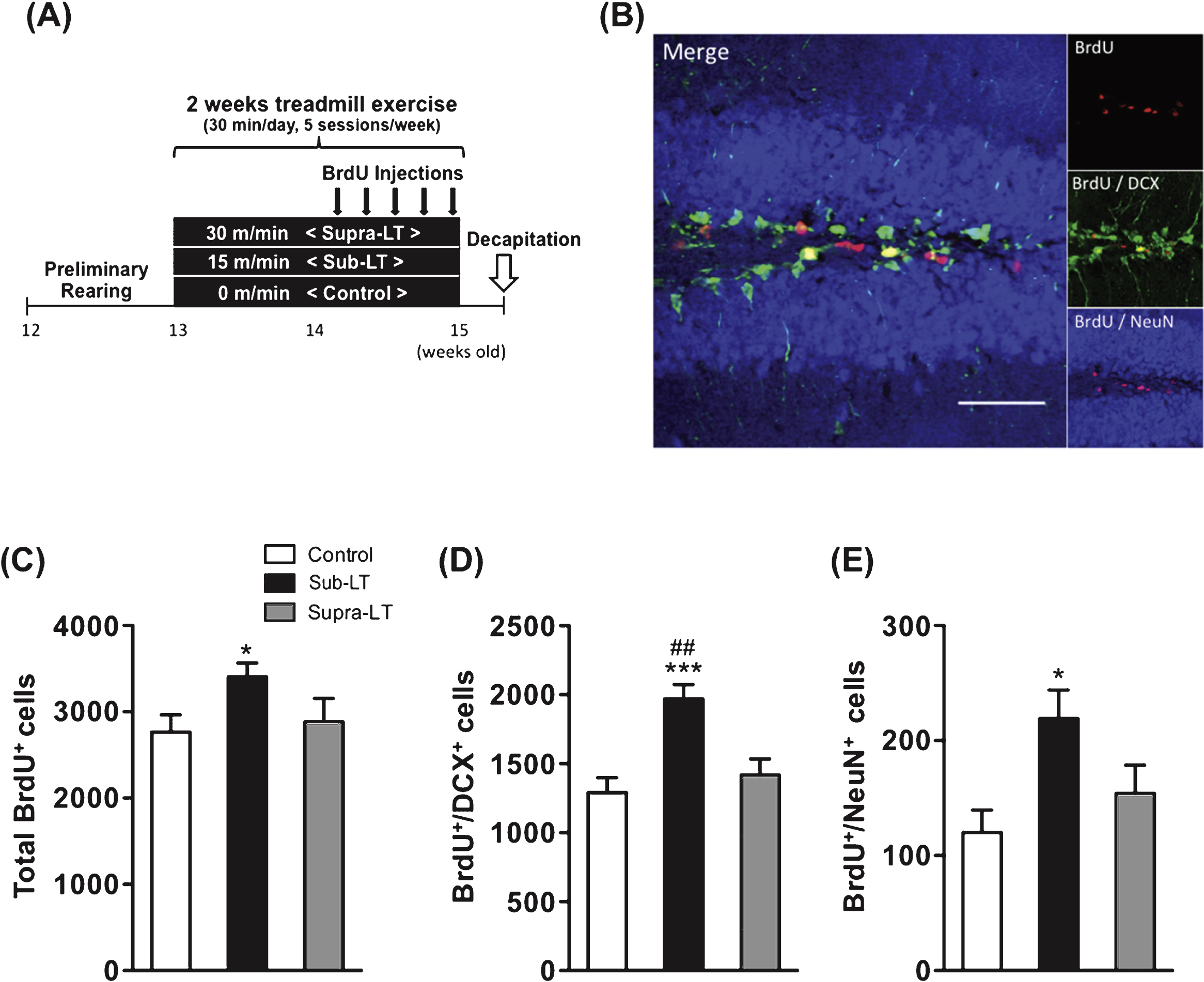
Fig.4
Effects of glucocorticoid receptor antagonists on exercise-induced adult hippocampal neurogenesis The role of glucocorticoid signaling was evaluated with the injection of the mineralocorticoid receptor (MR) antagonist spironolactone, and the glucocorticoid receptor (GR) antagonist mifepristone. (A) Total number of BrdU+ cells. There was a significant main effect of treadmill exercise (F (2,49) = 4.15, p < 0.05), but not glucocorticoid receptor antagonist (F (2,49) = 3.07, p = 0.06), and a significant glucocorticoid receptor antagonist×treadmill exercise interaction (F (4,49) = 4.7, p < 0.01). (B) Number of BrdU+/DCX+ cells. There was a significant main effect of treadmill exercise (F (2,49) = 5.59, p < 0.01), but not glucocorticoid receptor antagonist (F (2,49) = 3.72, p = 0.69), and a significant glucocorticoid receptor antagonist×treadmill exercise interaction (F (4,49) = 4.86, p < 0.01). (C) Number of BrdU+/NeuN+ cells in the dentate gyrus. There was a significant main effect of treadmill exercise (F (2,47) = 3.23, p < 0.05), but not glucocorticoid receptor antagonist (F (2,47) = 0.61, p = 0.55), and no significant glucocorticoid receptor antagonist×treadmill exercise interaction (F (4,47) = 1.87, p = 0.14). Data represent the mean ± SEM (n = 6 -7 mice). *, p < 0.05, **, p < 0.01, ***, p < 0.001 in comparison with respective control mice and #, p < 0.05, ##, p < 0.01 in comparison with supra-LT (two-way ANOVA and Bonferroni post hoc tests).
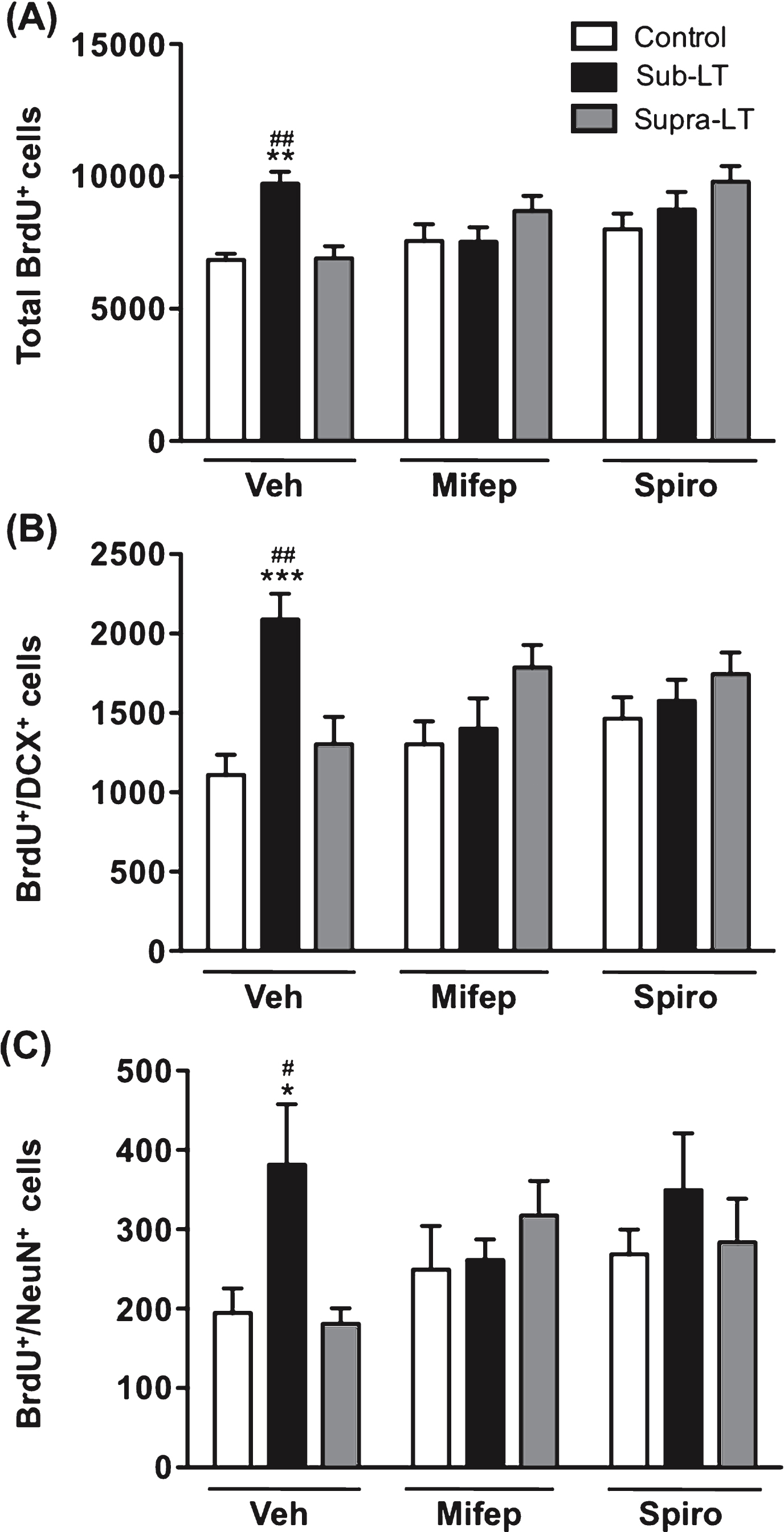
Table 1
Body weight, adrenal weight, adrenal/body weight ratio, corticosterone levels and DG volume in mice treated with glucocorticoid receptor antagonists
Vehicle | Mifepristone | Spironolactone | |||||||
Control | Sub-LT | Supra-LT | Control | Sub-LT | Supra-LT | Control | Sub-LT | Supra-LT | |
Body weight (g) | 28.8 ± 0.65 | 29.7 ± 0.80 | 29.6 ± 0.43 | 28.1 ± 0.63 | 28.0 ± 0.38 | 27.8 ± 0.17* | 29.0 ± 0.26 | 29.0 ± 0.52 | 29.0 ± 0.93** |
Adrenal weight (mg) | 3.7 ± 0.08 | 3.96 ± 0.18 | 4.03 ± 0.11 | 3.19 ± 0.15 | 3.51 ± 0.16 | 3.77 ± 0.14** | 3.42 ± 0.14 | 3.62 ± 0.11 | 4.16 ± 0.14** |
Adrenal/body weight ratio | 0.13 ± 0.004 | 0.13 ± 0.06 | 0.14 ± 0.002 | 0.11 ± 0.005 | 0.13 ± 0.005 | 0.14 ± 0.005 | 0.12 ± 0.005 | 0.13 ± 0.005 | 0.14 ± 0.001 |
Corticosterone (ng/dl) | 132.7 ± 26.0 | 185.3 ± 31.8 | 176.0 ± 40.3 | 106.2 ± 17.4 | 136.2 ± 26.6 | 199.2 ± 35.1 | 89.8 ± 19.3 | 101 ± 24.7 | 178 ± 14.7 |
DG volume (mm3) | 0.6 ± 0.01 | 0.58 ± 0.02 | 0.58 ± 0.02 | 0.57 ± 0.02 | 0.62 ± 0.03 | 0.58 ± 0.02 | 0.61 ± 0.03 | 0.62 ± 0.02 | 0.6 ± 0.02 |
Adrenal to body weight ratio of mice treated glucocorticoid receptor antagonists (mifepristone, spironolactone) was significantly increased with supra-LT treadmill exercise. There was a significant main effect of glucocorticoid receptor antagonists (F (2,45) = 2.7, p < 0.01), a significant main effect of treadmill exercise (F (2,45) = 11.03, p < 0.01), and a significant effect on adrenal weight with no glucocorticoid receptor antagonists×treadmill exercise interaction (F (4,45) = 0.92, p = 0.46). Data are mean ± SEM for 5–7 mice. *: p < 0.05, **: p < 0.01 in comparison to respective control mice (two-way ANOVA and Bonferroni post hoc tests).